Excerpt From Report RI 8507
RI 8507 is from the U.S. Bureau of Mines Reports
STRUCTURE RESPONSE AND DAMAGE PRODUCED BY
GROUND VIBRATION FROM SURFACE MINE BLASTING
D. E. Siskind, Geophysicist,
M.S. Stagg, Civil Engineer
J. W. Kopp, Mining Engineer
Twin Cities Research Center, Bureau of Mines, Twin Cities, Minn.
C. H. Dowding, Civil Engineer; Professor of Civil Engineering. Northwestern University,
Evanston, Ill.
ABSTRACT
The Bureau of Mines studied blast-produced ground vibration from surface mining to assess its damage and annoyance potential, and to determine safe levels and appropriate measurement techniques. Direct measurements were made of ground-vibration-produced structure responses and damage in 76 homes for 219 production blasts. These results were combined with damage data from nine other blasting studies, including the three analyzed previously for Bureau of Mines Bulletin 656.
Safe levels of ground vibration from blasting range from 0.5 to 2.0 in/sec peak particle velocity for residential-type structures. The damage threshold values are functions of the frequencies of the vibration transmitted into the residences and the types of construction. Particularly serious are the low-frequency vibrations that exist in soft foundation materials and/or result from long blast-to-residence distances. These vibrations produce not only structure resonances (4 to 12 Hz for whole structures and 10 to 25 Hz for midwalls) but also excessive levels of displacement and strain.
Threshold damage was defined as the occurrence of cosmetic damage; that is, the most superficial interior cracking of the type that develops in all homes independent of blasting. Homes with plastered interior walls are more susceptible to blast-produced cracking then modern gypsum wallboard; the latter are adequately protected by a minimum particle velocity of approximately 0.75 in/ sec for frequencies below 40 Hz. Structure response amplification factors were measured; typical values were 1.5 for structures as a whole (racking) and 4 for midwalls, at their respective resonance frequencies. For blast vibrations above 40 Hz, all amplification factors for frame residential structures were less than unity.
The human response and annoyance problem from ground vibration is aggravated by wall rattling, secondary noises and the presence of airblast. Approximately 5 to 10 pct of the neighbors will judge peak particle velocity levels of 0.5 to 0.75 in/sec as “less than acceptable” (i.e., unacceptable) based on direct reactions to the vibration. Even lower levels cause psychological response problems, and thus social, economic, and public relations factors become critical for continued blasting.
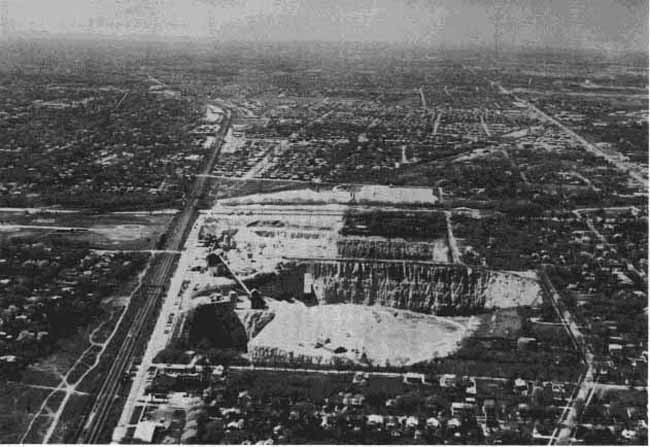
INTRODUCTION
Ground vibrations from blasting have been a continual problem for the mining industry, the public living near the mining operations, and the regulatory agencies responsible for setting environmental standards. Since 1930, the Bureau of Mines has studied various aspects of ground vibration, airblast, and instrumentation, culminating in Bulletin 656 in 1971(37).
In that publication, Nicholls extensively reviewed blast design effects on the generation of vibrations, ground vibration and airblast propagation, and seismic instrumentation. Bulletin 656 established the use of peak particle velocity in place of displacement, a minimum delay interval of 9 msec for scaled distance calculations, and a safe scaled distance design parameter of 50 ft/lb² for quarry blasting in the absence of vibration monitoring. The authors also included a damage summary analysis originally published in 1962 by Duvall and Fogelson as Bureau of Mines Report of Investigations 5968 (14). New data available since the 1962 report were described in Bulletin 656, but a new analysis to include these data was not performed.
Recommended was the use of peak particle velocity to assess the damage potential of the ground vibrations, and 2.0 in/sec as an overall safe level for residential structures. These recommendations have been widely adopted by the mining and construction industry and incorporated into numerous State and local ordinances that regulate blasting activity. Soon after publication of the 2.0-in/sec safe level criterion, it became apparent that it was not practical to blast at this high vibration level. Many mining operations with nearby neighbors were designing their blasts to keep velocities as low as 0.40 in/sec. Severe house rattling caused fear of property damage below the 2.0-in/sec level, and many homeowners were attributing all cracks to the blast vibrations.
Pennsylvania was the first State to adopt the 2.0-in/sec peak particle velocity criterion as a safe standard in 1957. However, in 1974 it was forced to adopt stricter controls because of citizen pressure and lawsuits involving both annoyance and alleged damage to residences. There existed no technologically based and supportable criteria for mine, quarry, and construction blasting other than the 2.0-in/sec criteria from Bulletin 656 and RI 5968. The general growth of mining, the proximity of mining and quarrying to their residential neighbors, and greater environmental awareness have all required reexamination of blasting regulations and justified further research.
In 1974 the Bureau of Mines began to reanalyze the blast damage problem, expand the Duvall and Fogelson 1962 study, and overcome its more serious shortcomings through the following efforts:
1. Direct measurements were made of structural response, and damage was observed in residences from actual surface-mine production blasting.
2. Damage data from six additional studies, not available in 1962, were combined with three studies analyzed by Duvall and Fogelson, plus the new Bureau of Mines measurements.
3. Probabilistic analysis techniques were used on various sets of data, as well as the conventional statistical derivation of mean square fit and standard deviation for the various damage thresholds.
4. Particular emphasis was placed on the frequency dependence of structure response and damage, recognizing that the response characteristics and frequency content of the vibrations are critical to response levels and damage probabilities.
5. An analysis was made of various studies of human tolerance to vibrations, although most data are from steady-state rather than impulsive sources.
An understanding of how houses respond to ground vibration and the vibration characteristics most closely related to this response will enable operators to design blasts to minimize adverse effects. The mining industry needs realistic design levels and also practical techniques to attain these levels. At the same time, environmental control agencies responsible for blasting and explosives need reasonable, appropriate, and technologically established and supportable criteria on which to base their regulations.
Finally, neighbors around the mining operations and other blasting, as shown in figure 1, require protection of their property and health so that they do not bear an unreasonable personal cost.
This report summarizes the state of knowledge on damage to residences from surface mine, quarry, and construction blasting. Included are discussions of applicable data on fatigue and human response, although work is continuing in these areas. An analysis was also made on vibration production from mining blasts. The generation and propagation data in Bulletin 656 are for smaller quarry blasts, which are also typically characterized by thin overburden layers.
The damage criteria presented herein were developed to quantify the response of and damage to residential-type structures from small to intermediate sized blasts as used in mining, quarrying, construction, and excavation. Application of these criteria by regulatory agencies will require an analysis of social and economic costs and benefits for the coexistence of blasting and an environmentally conscious society.
ACKNOWLEDGMENTS
The authors acknowledge the generous assistance of many regulatory agencies, engineering consultants, powder companies, homeowners, and mine and quarry operators. Special thanks are due to the Pennsylvania Department of Environmental Resources for demonstrating the need for this ground vibration research. Much of the fieldwork and data reduction was done by Virgil J. Stachura, Alvin J. Engler, Steven J. Sampson, Michael P. Sethna, Bryan W. Huber, Eric Porcher, and John P. Podolinski. Valuable technical support was provided by G. Robert Vandenbos for all stages of the blasting research.
GROUND VIBRATION CHARACTERISTICS
Ground vibrations from blasting are an undesirable side product of the use of explosives to fragment rock for mining, quarrying, excavation, and construction. This ground vibration or seismic energy is usually described as a time varying displacement, velocity, or acceleration of a particular point (particle) in the ground. It can also be measured as various integrated (averaged) energy levels. Three mutually orthogonal time-synchronized components are required to characterize the motion fully. Alternatively, the three components can be combined into a true vector sum for any instant in time or a pseudo vector sum derived from vector addition of the maximums of each component, independent of time (50).
The descriptors for motion are related by integration and differentiation (note that one should reference the original document for the accuracy of these equations):
V = (d/dt) D = ∫ Adt.
and A = (d/dt) V = (d²/dt²) D
where D is displacement, V is velocity, and A is acceleration. When the vibrations can be approximated by a sine wave (simple harmonic motion), the relationships above become:
D = Do sin(2πft),
V = Do (2πft)cos(2πft) = Vocos(2πft),
and A = -Do(2πft)²sin (2πft)
= – Aosin(2πft).
where f is frequency, t is time, and, Do, Vo, and Ao are constants. Peak values correspond to the time when the trigonometric functions equal unity, and the relationships for these peaks values then become:
Do = Vo/2πf = Ao/(2πf)²
Vo = 2πfDo = Ao/2πf
and Ao= (2πf)²Do = 2πfVo
Complex vibrations cannot be approximated by the simple harmonic motion, and either electronic or numeric (computer) integration and differentiation become necessary for conversions.
Interactions between the vibrations and the propagating media give rise to several types of waves, including direct compressional and shear body waves, refracted body waves, and both horizontally and vertically polarized surface waves. These vibrational waves are of primary importance in studies of the earth’s interior and earthquake characteristics, but their individual effects have been totally neglected in blasting seismology. Analysis of damage to structures does not require knowledge of what happens between the source and the receiver or of the type of wave. It requires only the vibrational input to the house at its foundation. Additionally, multiply delayed shots are sufficiently complex vibration sources to make identification of individual waves difficult, if not impossible, under most conditions.
TIME AND FREQUENCY PROPERTIES OF MINING BLASTS
The amplitude, frequencies, and durations of the ground vibrations change as they propagate, because of (a) interactions with various geologic media and structural interfaces, (b) spreading out the wave-train through dispersion, and/or (c) absorption, which is greater for the higher frequencies. Close to the blast the vibration character is affected by factors of blast design and mine geometry, particularly charge weight per delay, delay interval, and to some extent direction of initiation, burden, and spacing (56). At large distances the factors of blast design become less critical and the transmitting medium of rock and soil overburden dominate the wave characteristics.
Particle velocity amplitudes are approximately maintained as the seismic energy travels from one material into another (i.e., rock to soil), probably from conservation of energy. However, the vibration frequency and consequently the displacement and acceleration amplitudes depend strongly on the propagating media. Thick soil overburden as well as long absolute (as opposed to scaled) distances create long-duration, low-frequency wave trains. This increases the response and damage potential of nearby structures.
Frequencies below 10 Hz produce large ground displacement and high levels of strain, and also couple very efficiently into structures where typical resonant frequencies are 4 to 12 Hz for the corner or racking motions. Racking is whole structure distortion with characteristic shear stresses and failures. Previous studies described the frequency character of vibration from quarry (37) and coal mine blasts (56), and a recent report by Stagg on instrumentation for ground vibration summarized the frequency characteristics of vibrations from small to moderate-sized blast sources (50). Ground vibration frequencies from three types of blasts are shown in figure 2, all measured at the closest residence where peak particle velocities were within 0.5 to 2.0 in/ sec. Although the shot types in figure 2 are labeled coal mine, quarry, and construction, the frequency-determining factors are the shot sizes, distances, and rock competence. The coal mine and quarry blasts were all more than 200 lb/delay at distances exceeding 350 ft. The construction (and excavation) shots ranged from 1¼ to 12¾ lb at distances of 30 to 160 ft. Soil overburdens were 0 to 5 ft for construction, under 10 ft for quarries, and generally above 5 to 10 ft for coal mines.
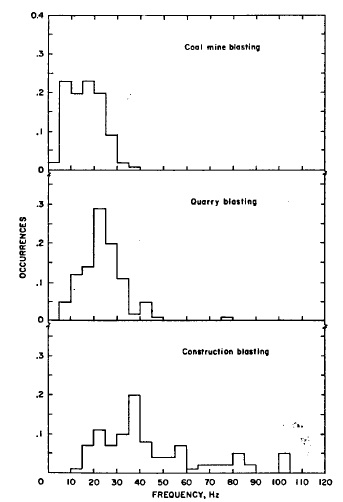
Time histories and Fourier frequency amplitude spectra from three typical blasts measured by a buried three-component transducer are shown in figures 3 to 5 (50). The coal mine shot is characterized by a trailing large-amplitude, low-frequency wave, which is probably a surface wave generated in the overburden layers. Quarry blasts do not usually show this low-frequency tail for one or more of the following reasons: smaller charge weights, smaller shot to instrument distances, and thinner soil overburdens. The combination of large shots, thick soil and sedimentary rock overburdens, relatively good confinement, and long-range propagation make coal mine blast vibrations potentially more serious than quarry and construction blasts because of their low frequencies. By contrast, coal mine highwall blasts are inefficient generators of airblast (46). Hard rock construction and excavation blasts tend to be shorter in duration and contain higher frequency motions than those of either coal mine or quarry.
Frequency characteristics of blast vibrations depend strongly on the geology and blast delay intervals. Except for the short-distance, all-rock case, they are difficult to predict and vary widely. Therefore, it is desirable to obtain complete time histories rather than simple peak values in any sensitive areas. Many examples of continual complaints about severe rattling at levels below 0.5 in/sec are attributable to the low frequencies. Research is continuing on the effects of blast design, face orientation, and near surface geology on the character of both the ground vibrations and airblast.
OTHER VIBRATION SOURCES
Earthquakes, nuclear blasts, and very large scale, in situ mining shots all produce potentially damaging ground vibrations, as well as do other static and quasistatic vibration sources (traffic, pile driving, sonic booms, etc.). The first Bureau of Mines blast vibration summary in 1942 examined the levels of earthquake vibrations and the corresponding Mercalli intensities for damage, and concluded these did not apply to blasting (51). Earthquakes produce long-duration and very low frequency events.
Acceleration levels are typically used by seismologists to quantify damage potential. These may be of moderate and even lower levels than found in blasting; however, their low frequencies produce large particle velocities and enormous displacements. As an example, Richter states that a 0.1 g acceleration at 1 Hz is ordinarily considered damaging in earthquake seismology (41). The corresponding particle velocity and displacement are 6.15 in/sec and 0.98 in, respectively, assuming simple harmonic motion. The same acceleration at 20 Hz would only produce 0.308 in/sec particle velocity and 0.0025 in displacement. Richter also observes that the damage potential of a given vibration is dependent on its duration, with 0.1 g at 1 Hz likely not to produce damage for events of a few seconds, but very serious for earthquake-type events of 25 to 30 sec (41).
A similar case is provided by the Salmon nuclear study and similar large blasts (5, 35, 39, 42-43, 45). These blasts all produced low-frequency and long-duration ground vibrations resulting from their sizes and distances. The Salmon vibration time history was 90 sec long at the structures ( 18 to 31 km) that were alleged to have been damaged. These durations are hardly comparable to those in mine, quarry, and construction blasting. Consequently, damage data of this kind cannot justifiably be correlated with the scale of blasting of concern in this analysis. However, the dynamic modeling techniques developed during the extensive research of earthquake and nuclear blast response can be applied to the study of blasting and the mechanisms of structural response.
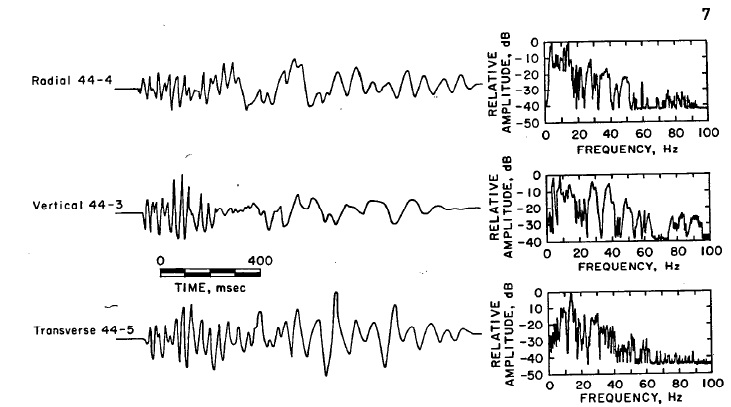
GENERATION AND PROPAGATION
Much research has been conducted on ground vibrations. Generation and propagation of ground vibrations have been studied extensively to determine the effects of blast design and geology on vibration amplitudes and frequency character. In Bulletin 656, Nicholls summarized the Bureau’s investigation of vibrations produced by blasting in 25 stone quarries, dating back to 1959 (37). The Bureau also conducted a series of studies of vibrations generated in four operating underground metal mines in 1974 (45). A major study was recently completed by Wiss that quantifies the influence of many of the blast design parameters on both ground vibration and airblast generation and propagation in five surface coal mines (56). Lucole also recently published the results of a year of routine monitoring of vibration levels generated by various types of blasting (29).
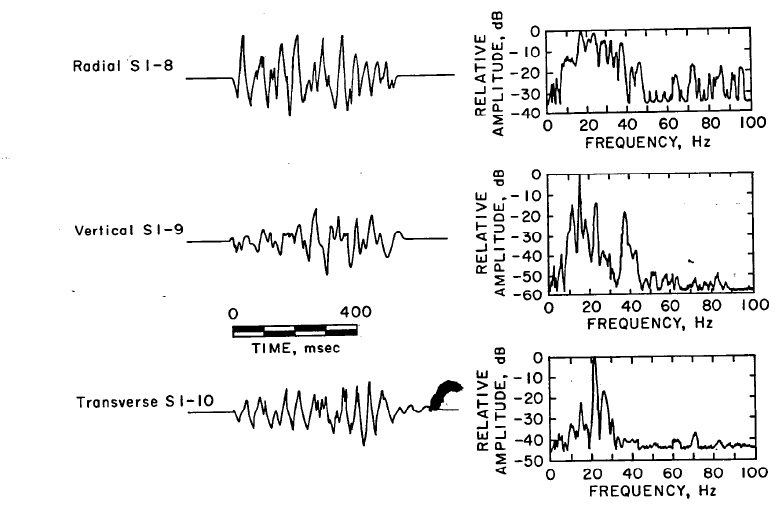
Prior to the last two studies, no data existed on vibrations generated by blasting in surface coal mines. It has been standard practice to apply the blast design rules developed for the small-hole, hard-rock quarry blasting to surface coal mines. Blast holes in surface coal mines have typical diameters exceeding 6 in, and in large area mines they are typically 9 to 15 in. These diameters are larger than those used in most quarries. The highwall blasts of surface coal mines are heavily confined, since they are used only to loosen the overburden and produce little or no throw. Decking is often used with complex timing systems, combining electronic and pyrotechnic delays. The rock being blasted is highly layered and of lower sonic velocity and strength than that in aggregate and lime quarries. Distances to houses are usually greater than for quarries, which are often in or near urban centers. Soil and incompetent rock overburden beneath structures near coal mines is normally tens of feet thick, far more than at most quarries.
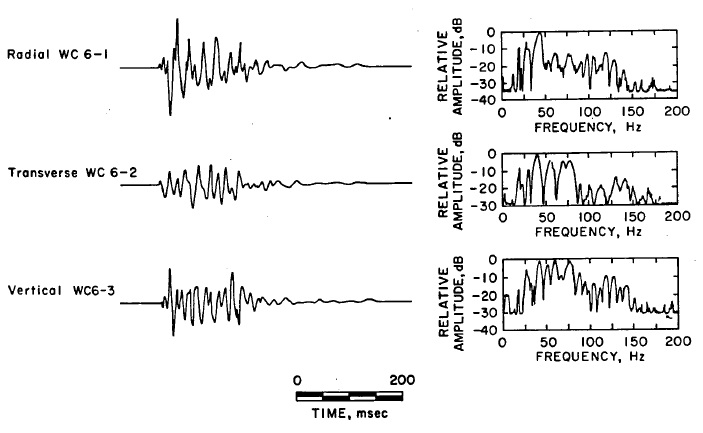
Consequently, coal mine blasting is normally characterized as follows:
1. Relatively large charge weights per delay.
2. Complex delay systems that are optimized for efficient fragmentation but that may produce adverse ground vibration frequencies.
3. Relatively high ground vibration levels close-in from heavy confinement of highwall shots.
4. Relatively rapid falloff of ground vibration levels with distance because of attenuation in weak rock.
5. Ground vibrations having predominantly low frequencies because of thick soil overburdens, strong geologic layering that favors surface waves, and large blast-to-structure distances.
BLAST DESIGN AND GROUND VIBRATION GENERATION
As in studies on quarry blasting, most blast design parameters for surface coal mine blasts have little influence on the generated vibrations. Charge weights per delay were again the most influential parameter. A small decrease in ground vibrations was noted for shallow as opposed to great depths of burial. Also, the location of the receiver relative to both the face and direction of blast initiation influenced the delay intervals at which constructive wave interference was experienced (56).
The Bureau of Mines vibration data are given in table 1. Included are charge weights, distances, ground vibration, and structure vibration levels for the predominantly coal mine blasts. The two horizontal components of motion were alined with the walls of the nearby structures for analysis of response and did not necessarily correspond to the traditional “radial” and “transverse.” The “structure number” of table 1 is for identification, and the “structure type” is the number of stories.
Vibration levels generated from one surface coal mine are shown in figure 6. The maximum horizontal and vertical ground motions were plotted for each blast. Equations and statistics for the various vibration propagations, including Site A (fig. 6), are given in table 2. All particle velocities are in inches per second, distances in feet, and charge weights in pounds. Propagation curves from a variety of surface coal mines are given in figures 7-9. Six of the propagation curves (Nos. 1-2 and 6-9) are from vertical hole blasts studied by Wiss (56). The remaining propagation curve (No. 19) is from a single Bureau of Mines site, where actual radial and transverse values were available.
Click below to see the original document in its entirety.