NCHRP 25-25/Task 72
CURRENT PRACTICES TO ADDRESS
CONSTRUCTION VIBRATION AND
POTENTIAL EFFECTS TO HISTORIC BUILDINGS
ADJACENT TO
TRANSPORTATION PROJECTS
Prepared by:
Wilson, Ihrig & Associates, Inc.,
ICF International, and
Simpson, Gumpertz & Heger, Inc.
September 2012
The information contained in this report is part of NCHRP Project 25-25, Task 72 National Cooperative Highway Research Program.
SPECIAL NOTE: This report IS NOT an official publication of the National Cooperative Highway Research Program, Transportation Research Board, National Research Council, or The National Academies. Note added by this website: The original document, as noted below, was created using money from Federal, State, and local governments. It has been changed from its original format to present on this page.
i
Acknowledgements
This study, Project 25-25 (Task 72), Construction Practices to Address Construction Vibration and Potential Effects on Historic Buildings Adjacent to Transportation Projects, was conducted with funding provided through the National Cooperative Highway Research Program (NCHRP). Annual voluntary contributions from state departments of transportation support the NCHRP. Project 25-25 (Task 72) is intended to fund quick response studies that are of interest to the highway design and construction community.
Richard A. Carman, Ph.D., P.E. (Principal Investigator) of Wilson, Ihrig & Associates, Inc. prepared this report with support from David Buehler, P.E. and Stephen Mikesell of ICF International, and Carolyn L. Searls, P.E. of Simpson, Gumpertz & Heger. Deborah A. Jue and Ani S. Toncheva of Wilson, Ihrig & Associates and Edward Yarbrough and Eva Hsu of ICF International also contributed to the project. Technical oversight and guidance was provided to this research team by a panel composed of members from state departments of transportation and a private consulting firm:
Dr. Gail Anne D’Avino, Georgia DOT
Mr. Karel Cubick, MS Consultants
Ms. Joyce McKay, New Hampshire DOT
Mr. Robert S. Newbery, Wisconsin DOT
Mr. Antony F Opperman, Virginia DOT
Mr. Mario Sanchez, Texas DOT
Mr. Timothy V. Sexton, Washington DOT
Mr. John Underwood, Mississippi DOT
Ms. Mary Ann Naber, Federal Highway Administration
Ms. Stephanie Stoermer, Federal Highway Administration
Ms. Elizabeth Zelasko Patel, Federal Transit Administration
Nanda Srinivasan, NCHRP Senior Program Officer, managed the project.
Disclaimer
The opinions and conclusions expressed or implied are those of the research agency that performed the research and are not necessarily those of the Transportation Research Board or its sponsoring agencies. This report has not been reviewed or accepted by the Transportation Research Board Executive Committee or the Governing Board of the National Research Council.
Instructions to Panel Members
This project is being conducted under NCHRP Project 25-25 (Task 72), Construction Practices to Address Construction Vibration and Potential Effects on Historic Buildings Adjacent to Transportation Projects. The report will not go through the usual rigorous review process established and monitored by the Transportation Research Board Executive Committee or the Governing Board of the National Research Council, and should not be described as a “TRB Report.” It should be described as a contractor’s report conducted with funding provided through National Cooperative Highway Research Program Project 25-25 (Task 72). NOTE: The Transportation Research Board of the National Academies, the National Research Council, the Federal Highway Administration, the American Association of State Highway and Transportation Officials, and the individual states participating in the National Cooperative Highway Research Program do not endorse products or manufacturers. Trade or manufacturers’ names appear herein solely because they are considered essential to the object of this report.
ii
Table of Contents
1. INTRODUCTION ………. 1
2. BACKGROUND ON VIBRATION AND DAMAGE ………. 1
2.1 Terminology ……….1
2.2 Construction Activity ……….2
2.3 Vibration Propagation ……….2
2.4 Building Response and Damage ……….2
2.5 Building Settlement ……….3
3. SECTION 106 PROCESS ……….3
3.1 Section 106 and Vibration ……….3
3.2 The Section 106 Process in General ……….3
3.2.1 Initiating the Process ……….4
3.2.2 Identifying the Area of Potential Effects and Historic Resources ……….4
3.2.3 Assessing Effects ……….4
3.2.4 Resolving Adverse Effects ……….5
3.3 Section 106 as it Applies to Highway Construction Vibration ……….6
4. LITERATURE REVIEW ……….6
4.1 Vibration Criteria ……….8
4.2 Current Practices ……….10
4.3 Procedures ……….10
4.4 Human Perception of Vibration ……….10
4.5 Summary ……….12
5. TRANSPORTATION AGENCY SURVEY ……….13
5.1 Survey Response ……….14
5.2 Follow-Up Calls to Survey Respondents ……….14
6. CASE STUDIES ……….15
6.1 Case Study #1: Sacramento Railyards Central Shops (Carman, et al.) ……….16
6.2 Case Study #2: Cypress Lawn Cemetery – Entrance Archway and de la
Montaña Mausoleum ……….18
6.3 Case Study #3: Gipfel Brewery Building, Milwaukee (HNTB Corporation
and Wilson, Ihrig and Associates) ……….21
6.4 Case Study #4: Fraunces Historic District, New York City
(Esrig and Ciancia) ……….23
6.5 Case Study #5: Fort Point – San Francisco ……….24
6.6 Case Study #6: Historic District, New York City (Hammarberg, et al.) ……….26
6.7 Case Study #7: Presidio Buildings – Doyle Drive, San Francisco (ICF International) ……….27
iii
7. RECOMMENDATIONS ……….30
7.1 Assessment Approach ……….30
7.2 Research and Approach to Disseminate Research Findings ……….31
8. CONCLUSION ……….31
9. REFERENCES ……….32
Appendices
Appendix A. Literature Review
Appendix B. Agency Survey
Appendix C. Analysis Flow Chart
Tables
Table 1. Vibration Source Levels for Construction Equipment ……….9
Table 2. Vibration Source Levels for Construction Equipment ……….30
Figures
Figure 1. Typical Vibration Amplitudes and Thresholds ……….12
Figure 2. Car Shop within the Sacramento Railyards Central Shops Historic District ……….17
Figure 3. Cypress Lawn Cemetery Grand Gateway ……….19
Figure 4. Cypress Lawn Cemetery de la Montaña Mausoleum ……….20
Figure 5. Gipfel Brewery building being moved ……….23
Figure 6. Fraunces Tavern ……….23
Figure 7. Fort Point and Golden Gate Bridge ……….25
Figure 8. Buildings at the Presidio ……….28
iv
Acronyms and Abbreviations
AASHTO American Association of State Highway and Transportation Officials
ACHP Advisory Council on Historic Preservation
ADC40 Committee on Transportation Noise and Vibration
APE Area of Potential Effect
APTA American Public Transit Association
BART Bay Area Rapid Transit
BETP Built Environment Treatment Plan
Caltrans California Department of Transportation
CFR Code of Federal Regulation
CIDH cast-in-drilled-hole
DOTs Departments of Transportation
EIS Environmental Impact Statement
FHWA Federal Highway Administration
MOA Memorandum of Agreement
MOU Memorandum of Understanding
NCHRP National Cooperative Highway Research Program
NHL National Historic Landmark
NHPA National Historic Preservation Act
NRHP National Register of Historic Places
PHA Project Historical Architect
PPV Peak particle velocity
SHPO State Historic Preservation Officer
THPO Tribal Historic Preservation Officer
TRB Transportation Research Board
1
1. INTRODUCTION
NCHRP identified a need for a comprehensive compilation of current and successful practices that address construction vibration impacts on historic buildings adjacent to roadway construction projects. The goal of this compilation is to help historic preservation resource agencies and organizations, departments of transportation (DOTs), and the public understand the technical aspects of vibration impact studies.
To prepare the compilation of current and successful practices, the research team conducted a review of literature authored in the United States and abroad to identify the current state of the art for assessing the fragility of historic structures and their susceptibility to damage, for monitoring vibration transmission from construction projects, and for mitigating potentially damaging vibration.
The research team also surveyed state DOTs and other agencies to understand how they currently address this issue and to identify several case studies that illustrate how construction vibration effects on historic buildings have been recently evaluated.
This report summarizes the results of the literature search and the survey of transportation agencies and provides a detailed discussion of seven informative case studies. A recommended guideline approach for addressing construction vibration effects on historic buildings has also been provided.
2. BACKGROUND ON VIBRATION AND DAMAGE
2.1 Terminology
The following discussion provides basic information on how construction-induced vibration is generated and measured. More information on terminology is provided in Appendices A and B.
Operation of heavy construction equipment, particularly pile drivers and other impact devices such as pavement breakers, creates seismic waves that radiate along the surface of the earth and downward into the earth. These surface waves can be felt as ground vibration. Vibration from operation of this equipment can result in effects ranging from annoyance of people to damage of structures.
As seismic waves travel outward from a vibration source, they excite the particles of rock and soil through which the waves pass and cause the particles to oscillate. The actual distance that these particles move back and forth is usually only a few ten-thousandths to a few thousandths of an inch. The rate or velocity (in inches/sec) at which ground particles oscillate varies from low values that are barely measureable (0.001 inches/sec) to values that can be more than 5 inches/sec. This maximum velocity value, referred to as the peak particle velocity (PPV), is a commonly accepted descriptor for ground vibration amplitude.
2
2.2 Construction Activity
Construction that can result in significant levels of ground vibration generally falls into two categories that best are characterized by the cause of the vibration and its duration. Vibration that is steady-state and more or less continuous can be caused by vibratory compaction of soil, vibratory pile driving, movement of large equipment, and other sources. In contrast, vibration that is much more transient in nature and intermittent due to impulsive forces can be caused by pile driving and blasting. Researchers on vibration and its effects on older, more fragile buildings generally indicate which type of vibration they are evaluating, but often do not distinguish between the two nor indicate that the type of vibration can have different effects on a structure. It is important to keep the two types of vibration in mind when setting vibration limits.
2.3 Vibration Propagation
Vibration travels through the ground from the point at which energy is imparted (e.g., blast), spreading out and getting reflected from different soil layers. As it travels, vibration attenuates because of the spreading and damping properties of the soil or rock through which the vibration travels. Consequently the process of vibration propagation is often complex and difficult to predict for any given site. However, some sites have enough empirical data available for use in predicting vibration away from the source.
2.4 Building Response and Damage
The manner in which a particular building will respond dynamically to strong ground vibration depends on many factors, among which are the soil on which the building is founded, the building’s foundation (e.g., spread footing, piles), the building’s mass, and the stiffness of the building’s main structural elements. Whether dynamic motion will damage the building’s structure and its architectural features (e.g., interior surface finishes) depends in large part on the type of construction (e.g., masonry) and the elastic behavior of the building material at higher levels of strain. Wood and steel are more elastic than masonry, such as brick and stone. Interior finishes that are more susceptible to damage are those such as lath and plaster. The condition of a building and its maintenance are important factors when assessing susceptibility to vibration damage and must be taken into account when setting vibration limits.
A modern categorization of damage, which is contained in BS Standard 7385: Part 1: 1990 (BSI, 1990) and ISO 4866-2010 (ISO, 2010), follows. Note that dusting of cracks may occur even when no cosmetic damage has been observed.
Cosmetic: The formation of hairline cracks on drywall surfaces or the growth of existing cracks in plaster or drywall surfaces; formation of hairline cracks in mortar joints of
brick/concrete blocks.
Minor: The formation of large cracks or loosening and falling of plaster or drywall surfaces, or cracks through bricks/concrete blocks.
Major: Damage to structural elements of the building, cracks in support columns, loosening of joints, splaying of masonry cracks, etc.
In these documents, the term “threshold damage vibration level” is defined as the highest vibration level at which no cosmetic, minor, or major damage occurs.
3
2.5 Building Settlement
The study conducted by the research team is focused on the direct shaking effects of construction-generated ground vibration on historic buildings. In a situation where the soil has high liquefaction potential, construction vibration can cause ground settlement or shifting that significantly reduces support provided by the soil. Rather than vibration itself, this ground settlement or shifting of the ground can cause damage to the building. This situation requires a geotechnical engineer and a review of analysis, mitigation, and monitoring processes that is beyond the scope of this study.
3. SECTION 106 PROCESS
3.1 Section 106 and Vibration
The National Historic Preservation Act (NHPA) establishes a process to evaluate potential effects on historic properties that may be caused by federally-funded or approved projects. This process would apply to potential damage to historic properties caused by construction vibration. The following discussion summarizes the NHPA process and how the process might apply to damage caused by construction vibration.
3.2 The Section 106 Process in General
The process to minimize potential harm and damage to historic properties outlined in the NHPA is commonly referred to as the Section 106 process. Section 106 mandates that each responsible federal agency “take into account the effect” of its project on historic properties. An “historic property” is any property that is listed in or qualifies for listing in the National Register of Historic Places (NRHP). Most commonly, these properties are buildings or archaeological sites.
The NHPA also creates the Advisory Council on Historic Preservation (ACHP) and empowers it to administer the Section 106 process. The ACHP published detailed regulations in 36 Code of Federal Regulation (CFR) Part 800, which lays out the Section 106 process. The NHPA also established the position of State Historic Preservation Officer (SHPO) and created the NRHP. Each SHPO is primarily responsible for implementing the Section 106 process in their state. The law also creates a position of Tribal Historic Preservation Officer (THPO), who has SHPO-like duties and powers on tribal lands.
The ACHP regulations lay out a four-step process for “taking into account” impacts on historic properties:
1. Initiating the process.
2. Identifying the area of potential effect and historic resources therein
3. Assessing effects.
4. Resolving adverse effects.
These steps are discussed in detail below. Most projects typically involve only the first three steps. It is only when there is an adverse effect that all four steps will be taken. The agency responsible for the undertaking leads the process. The SHPO or THPO is consulted during all four steps.
4
3.2.1 Initiating the Process
The first step is the simplest of the four. The agency must decide whether its project, or “undertaking,” is subject to Section 106. Certain types of activities, such as planning efforts, are not subject to Section 106 because they have no potential to cause harm to an historic property. It can be assumed, however, that any construction-related activity that is federally permitted or funded is subject to Section 106.
3.2.2 Identifying the Area of Potential Effects and Historic Resources
The second step involves determining whether there is an historic property within the area that might be affected by the project. The Part 800 regulations use the term “area of potential effects,” commonly called the Area of Potential Effect (APE), to refer to the impact zone.
The first step in the identification process is defining an APE, which is usually delineated on a map. The APE can differ from one type of project to the next depending on the undertaking. Defining the APE can be especially critical in assessing effects associated with construction related the geographic area or areas within which an undertaking may directly or indirectly cause alterations in the character or use of historic 36 CFR 800.16 (d)). The APE for a highway construction project typically encompasses the actual construction zone plus adjacent buildings, recognizing that construction may cause visual and auditory impacts beyond the actual construction zone. In many cases, those adjacent buildings could also be subject to damaging construction vibration impacts. It is possible, however, that the APE for vibration impacts would be different than the APE for visual impacts. The vibration-related APE should be defined on the basis of good science for predicting the area in which such impacts would likely occur.
A second sub-step within the identification process is to determine whether any of the properties within the APE meet the definition of “historic property” under Section 106. With many projects, there are properties that qualify for listing in the NRHP but which have not been formally evaluated. In such cases, the project proponent typically hires a preservation consultant or relies upon qualified staff to determine whether these properties meet the eligibility criteria necessary for listing in the NRHP.
In the many cases, the process ends at this point because there are no historic properties within the APE. When it is known that an historic property is located within the project’s APE, however, the agency would move to the third step, assessing effects.
3.2.3 Assessing Effects
In this step, the responsible agency must make a finding as to whether the project will have an effect on an historic property. Effect is defined as “alteration to the characteristics of an historic property qualifying it for inclusion in or eligibility for the National Register” (36 CFR 800.16 (i)). The regulations allow for three types of findings: no historic properties affected; adverse effect; and no adverse effect.
A finding of no historic properties affected is used in two ways: there are no historic properties within the APE; or the historic properties within the APE will not be affected in any manner.
5
A finding of adverse effect is a conclusion that the project will cause an adverse effect on an historic property within the APE. Adverse effect is defined as an action that would “alter, directly or indirectly, any of the characteristics of an historic property that qualify the property for inclusion in the National Register in a manner that would diminish the integrity of the property’s location, design, setting, materials, workmanship, feeling, or association” (36 CFR 800.5 (a) (1)).
A finding of no adverse effect is used in situations in which an effect will occur but the effect is not so severe that it meets the definition of an adverse effect. This finding is common in projects that involve repair or rehabilitation to an historic property. The rehabilitation work will “affect” the historic building but that effect will not be adverse if the rehabilitation is consistent with accepted historic preservation standards, as stated in the Secretary of the Interior’s Standards for Treatment of Historic Properties (36 CFR part 68).
Potential effects from vibration could be determined to be adverse depending upon the projected severity of damage to an historic building. If an engineering analysis indicated that vibration from highway construction would have a high likelihood to cause a building to suffer structural damage, that result would obviously be an adverse effect. However, the vibration projected by the engineer as likely to cause cracking (cosmetic damage changing the building’s appearance), but not weakening the structure might be seen as a lesser, but still adverse, effect. The resolution of potential adverse effects depends on many factors, which are discussed next.
3.2.4 Resolving Adverse Effects
This step is taken only when there is a finding of adverse effect. In this step, the responsible agency consults with the SHPO, the ACHP (if the ACHP chooses to participate), and with other consulting parties to arrive at steps that would reduce the adverse effect to an acceptable level or would mitigate for the adverse effect. Following this consultation, the various parties will memorialize the terms of their agreement in a Memorandum of Agreement (MOA).
There is an almost limitless range of terms for an MOA, depending upon the nature of the potential adverse effect. In a situation where construction of a project would require removal of an historic building, the MOA might specify that the building be recorded photographically prior to its destruction. This is one of the most commonly utilized options for mitigation of adverse effects on historic properties. However, because construction activity that causes vibration severe enough to destroy a building would be necessary only in an extremely rare case, it is unlikely that this mitigation measure would ever be applicable to vibration impacts.
Although damage to an historic building would be considered an adverse effect, this could be mitigated through a commitment to repair the damage in accordance with the Secretary of the Interior’s Standards. A responsible agency, for example, could make a finding that the project would likely cause minor damage to an historic building, but the agency would commit itself to using the Secretary of the Interior’s Standards in repairing that damage.
It might also be possible to move the building out of harm’s way. However, this may not be desirable because a building that is susceptible to vibration damage could be damaged through a move as well. Moreover, most preservationists do not approve of moving historic buildings because relocation removes the buildings from their context. In addition, moving a building automatically delists it from the NHRP, unless the move was previously approved by the Keeper
6
of Register. One of the case studies discussed herein involved an historic building that was eventually moved, but for reasons unrelated to construction of the transportation project.
In a situation where damage could occur, the MOA might specify appropriate repair work, using the Secretary of the Interior’s Standards. In a situation where potential damage can be avoided by implementing alternative construction methods, the MOA could specify the use of the alternative methods and require monitoring during construction to ensure that vibration does not exceed acceptable levels.
3.3 Section 106 as it Applies to Highway Construction Vibration
There are several conclusions that may be drawn about how Section 106 applies to highway construction-related vibrations. First, the Section 106 process applies to most highway construction projects because a high percentage of highway work is federally-assisted. If a project is not federally-assisted, it may nonetheless be reviewed under state or local historic preservation laws.
Second, there is a need for good science in helping to establish the vibration APE for a project. In other words, how wide is the geographic area in which vibration damage would likely occur? The answer to this question should be provided by technical experts and be based upon sound scientific analysis. In this respect, it is generally easier to analyze vibration impacts than visual impacts because visual analysis will always involve a degree of subjectivity and is not amenable to scientific analysis. Vibration impact analysis, on the other hand, can be based primarily on a quantitative, objective analysis. However, given the state of the art, determining vibration impact significance and mitigation still involves some subjectivity.
Third, the complexity of the Section 106 process will be in direct proportion to the severity of potential vibration impact. If the impact is so severe that the building may suffer structural damage, the SHPO and others may extend consultation and request that the project be redesigned to avoid the impact. Lesser impacts, however, could be resolved through a commitment to use alternative construction methods that generate less vibration or to use the Secretary of the Interior’s Standards as a guideline to repair or reinforce the building. Qualified preservation professionals will be required to assist in identifying historic properties, evaluating potential effects and developing mitigation or repairs to affected historic properties.
4. LITERATURE REVIEW
The research team conducted a literature search to gather the most recent information available on the subject. Using the information contained in a recent document as a starting point, further research was conducted using various on-line search engines (e.g., Google Scholar). The followup phone calls to certain individuals who responded to the questionnaire survey (described in Section 5) also produced additional reference material. A memo, summarizing the results of the literature review, is contained in Appendix A. A brief summary of the literature review is provided below.
During the literature search, the research team obtained several references that were published subsequent to the 2002 document (HNTB Corporation and Wilson, Ihrig and Associates). Including previous literature and newly obtained literature, the research team assembled 64 reference documents, including published journal articles, project reports, national and
7
international standards, government guidelines, government regulations, and books related to the subject.
After reviewing existing reference lists and conducting an on-line search, the Team reviewed the material obtained for the level of relevance to the research topic. To assist with this task, the research team devised a rating scheme that focused on the relevance of the material. This rating can also serve as a simple guide to users of this document who desire to go beyond the summary provided herein and read the full text of the reference literature for additional details.
In order to focus the review of the literature, a list of general subject matter was developed. The list consisted of essential elements of the research. The Team members identified fourteen (14) relevant areas or content categories for characterizing the material found in the literature. The categories are:
-
Historic, old or fragile buildings or other such structures are discussed
-
Construction (general and blasting) vibration effects are discussed
-
Damage is discussed
-
Protection from construction vibration (mitigation) is discussed
-
Ambient vibration effects are discussed
-
Vibration limits to protect structures are presented
-
A formal or structured process (government or otherwise) to evaluate and protect historic structure is discussed
-
A detailed case study is presented
-
Actual damage (or no damage) correlated to vibration is presented
-
Detailed construction vibration mitigation measures are presented
-
The fragile nature of structures is discussed (what makes a structure damage prone)
-
Prediction of vibration from construction is presented
-
Blasting vibration effects are discussed
-
Discussion of the potential for soil settlement
Using the above categories it was possible to systematically review the documents obtained and evaluate their applicability to the subject matter based on how thorough each one was in covering the identified areas of interest.
From the research team’s literature review, it has been concluded that there is consensus on many issues relating to protecting historic structures. The research team also concludes that there is no consensus concerning appropriate vibration limits. Most of the research in the field of building damage comes from blasting vibration and its effect on modern structures. The research team observed that blast effect researchers tend to recommend higher levels of vibration than researchers whose studies involve lower levels of vibration such as that associated with roadway traffic. There is very little research pertaining to the subject of common construction vibration.
It is unlikely that additional government research on construction vibration will happen any time soon. In the meantime it will probably be necessary to adopt a cautious approach to setting limits and allow for flexibility on a case-by-case basis. This seems like the most viable approach to take and seems to have some support based on the literature reviewed. The procedures for documenting the existing condition of buildings and monitoring vibration are well established and generally there is universal agreement on these procedures.
8
Many state DOTs have procedures for controlling and monitoring blast vibration. Aside from this, the State of California is the only DOT which has produced a set of detailed procedures for controlling general construction vibration associated with transportation projects. Neither the survey of state DOTs nor the follow-up phone calls revealed procedures similar to those of California. The details of the literature search and review can be found in a summary memo in Appendix A.
4.1 Vibration Criteria
Table 1 summarizes the range of vibration limits recommended by researchers, practitioners, and government standards for avoiding damage to historic buildings as obtained from the generally available literature. Only those limits that are unique and originated with the reference document are indicated.
Clearly there is a wide range of opinion on appropriate vibration limits for historic buildings and structures. At one end of the range is a conservative limit of 0.10 inches/sec except in the case of ancient ruins where 0.08 inches/sec is considered appropriate by some. At the other end of the range, some would consider 0.50 inches/sec or even 2.0 inches/sec to be appropriate.
Much of the research indicates that as the vibration frequency increases, building elements are better able to withstand higher levels of vibration. A full explanation of this phenomenon would require a rather lengthy discourse on structural dynamics. Suffice it to say that the reason has to do with the movement of main building elements (primarily walls) when subjected to base excitation. At lower frequencies, walls tend to deform more (the relative movement of different points on the wall), thus subjecting the brittle materials (in the case of masonry construction) from which they are made (such as brick and mortar) to higher stresses and strains. Based on the research, it would appear that using a frequency-based limit is probably the most reasonable approach. The German standard DIN 4150-3 is a good example of this approach.
Some case studies suggest that it is possible to set conservative vibration limits and still allow for some flexibility in modifying those limits based on detailed engineering investigation and analysis done on a case-by-case basis prior to award of the construction contract. Alternatively, the transportation funding agency could adopt conservative criteria and allow for flexibility after the award of contract based on detailed investigations to be conducted by the contractor, who would need to demonstrate based on an engineering analysis the appropriateness of higher limits.
9
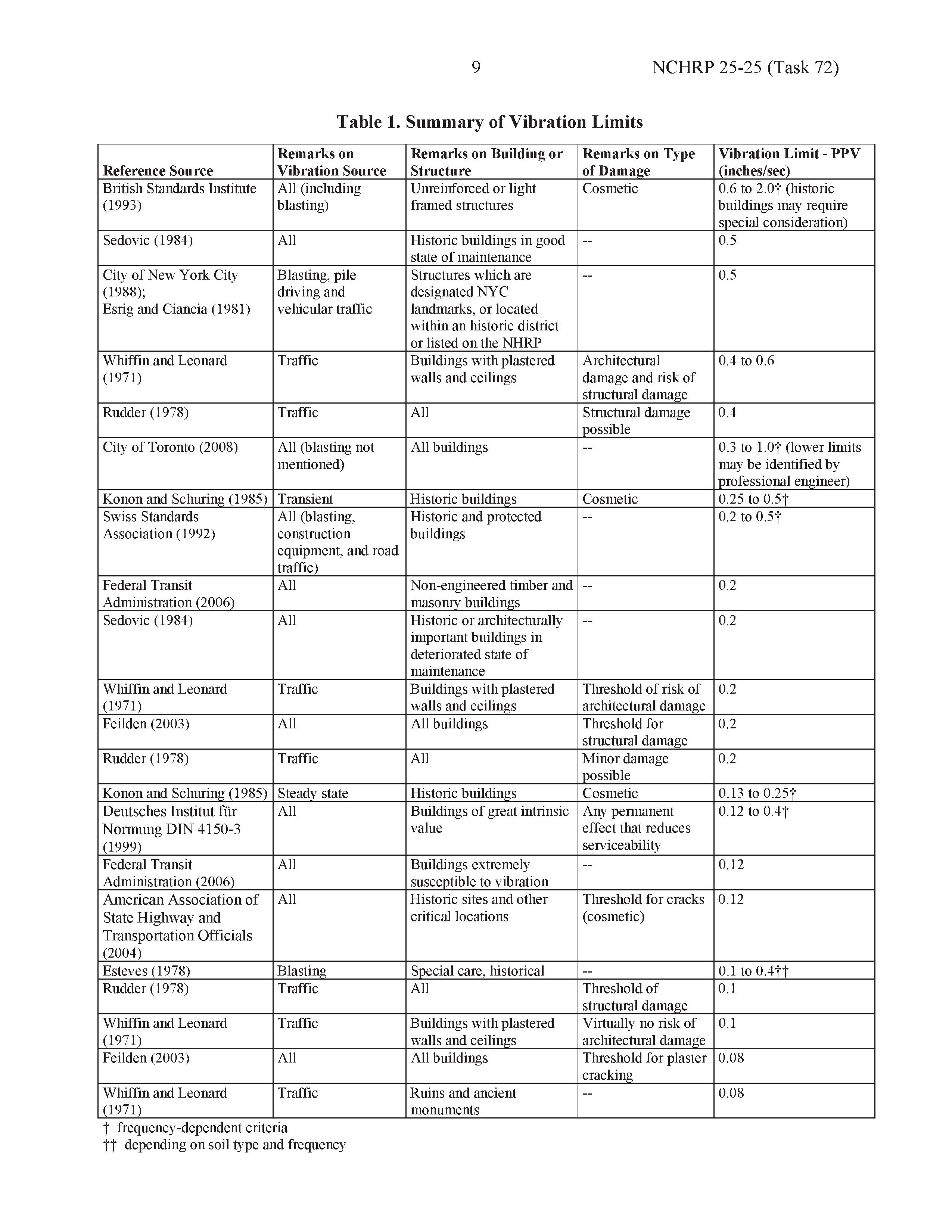
10
4.2 Current Practices
Current practices for controlling construction vibration affecting historic structures vary considerably. Most jurisdictions recognize a need to document the condition of the affected buildings prior to starting construction. This can be accomplished with still photo and video documentation. Where vibration limits are specified, vibration monitoring is required. Some agencies require warning thresholds to indicate when vibration levels are approaching the allowed limit. With current monitoring instrument technology, exceeding a threshold can be used to trigger a visual or audio alarm as well as an email notification of the event.
Incorporating a Project Historical Architect (PHA) into the process allows a professional trained in building preservation to work with the resident engineer in the decision making process when vibration limits are exceeded. Most mitigation measures included in project contract documents tend to be generic but do provide some means and methods that contractors can follow when vibration limits are exceeded.
4.3 Procedures
There is general agreement on the procedures to follow when dealing with construction vibration. The following are generally recommended steps to follow:
-
Consultation between historic building owner, development team and reviewing agencies, such as SHPO and local planning departments, to identify potential risks, negotiate changes, and agree on protective measures.
-
Documentation of building conditions prior to commencement of adjacent work, including a detailed photo survey of existing damage.
-
Establishment of vibration limits based on building conditions, founding soil conditions, and type of construction vibration.
-
Implementation of vibration mitigating measures on the construction site and/or at the historic building, which could include specific means and methods or protective measures.
-
Vibration monitoring during construction using seismographs, with notification by audible and/or visual alarms when limits are approached or exceeded.
-
Regular condition surveys and reviews during construction to identify damage, to evaluate the efficacy of protective measures already in place, and to identify and implement additional corrective steps.
4.4 Human Perception of Vibration
Although it was not the intent of this project to explore human reaction to construction vibration, it is important to address if only briefly. The potential effect of vibration on occupants of an affected building can have a substantial effect on the public’s reaction to a project. The vibration criteria presented by researchers discussed herein deal exclusively with the effects on buildings. However, a few documents included in the literature search also address human perception as well. Examples can be found in Caltrans (2004) and Whiffin and Leonard (1971). In addition the research team has relied on its experience from previous projects. Note that vibration measured at ground level can sometimes be lower than vibration inside the building due to amplification of vibration caused by resonances in building floors.
11
For continuous (steady state) vibration (e.g., vibratory compaction, vibratory pile driving):
-
PPV that exceeds 0.035 inches/sec is generally considered to be distinctly perceptible.
-
PPV of 0.10 inches/sec would be strongly perceptible and, according to Whiffin and Leonard (1971), begins to annoy.
-
PPV of 0.2 inches/sec is definitely annoying.
-
PPV between 0.4 and 0.6 inches/sec would be unpleasant, according to Whiffin and Leonard (1971).
Consequently, if one adopted a vibration limit of greater than 0.1 inches/sec for continuous vibration, a reaction from building occupants should be expected. The greater the limit, the greater the reaction would be.
Humans are generally considered to be less sensitive to transient (generally impulsive) vibration (e.g., impact pile driving, blasting), than to similar vibration from continuous sources. Kelly, et al. (1998) and Wiss (1981) present data that relate human perception to transient vibration as well as steady state vibration. For transient vibration (e.g., pile driving, blasting):
-
PPV between 0.04 and 0.2 inches/sec is considered to be barely perceptible.
-
PPV between 0.2 and 0.8 inches/sec would be distinctly perceptible.
-
PPV between 0.8 and 2.0 inches/sec should be strongly perceptible.
Comparing the two sets of data (continuous and transient), it can be seen that there is approximately a factor of eight difference in the sensitivity to the nature of the vibration.
Figure 1 provides a comparison between typical vibration levels at 25 feet from various common sources and the response of humans (annoyance) and the potential for damage to historic buildings. The sensitivity of humans makes them aware of vibration at levels much lower than those that result in damage to most contemporary structures, although older historic buildings can be at risk in the transition range of vibration that is just barely perceptible (0.02 inches/sec) to vibration that most people would find definitely annoying (0.2 inches/sec).
12
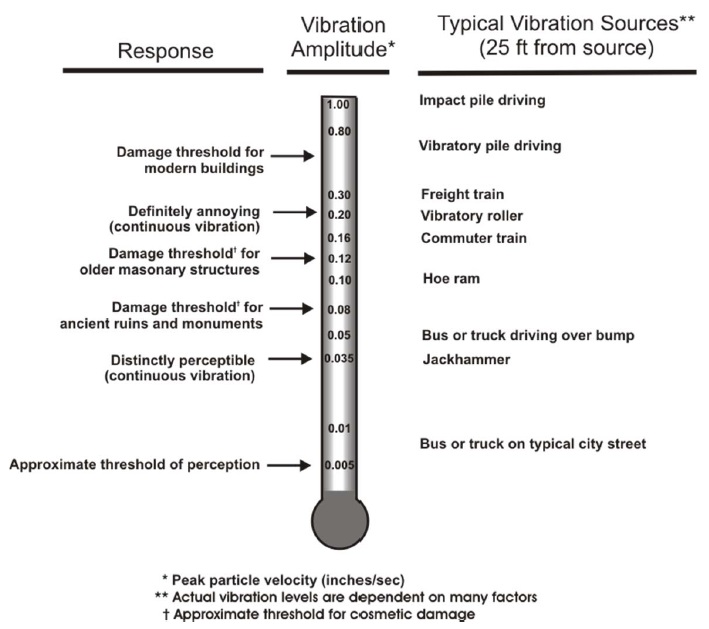
Agency staff and consultants who interact with the public and building owners in particular should be aware of potential human reaction to vibration. They should be prepared to explain in terms that can be understood by the average person that just because a person can feel vibration does not automatically mean that damage is occurring, They also should be prepared to explain that even though older buildings tend to be more susceptible to vibration, adequate measures can be implemented that protect against damage.
4.5 Summary
Recommended vibration limits tend to vary considerably within the published literature and national standards. The research team attributes this to the viewpoint of the researcher preparing the recommendation. The primary variables affecting the recommendation appear to be whether the field research was focused on blasting (at the high end of vibration) or motor vehicle traffic (at the low end of vibration), with the differences between these two types of vibration being the time history of the vibration (i.e., transient vs. continuous) and the number of vibration cycles to which a building is subjected.
13
The background of the researcher also appears to have some effect on the recommended limits as well. Researchers from the preservation profession tend to adopt a more conservative approach than researchers in the engineering profession, which is not unexpected. While some older buildings may be more susceptible to damage than new construction, the determining factors are the construction type and condition, not whether the building is historic. Human reaction to construction vibration might also be a consideration when setting limits or at least taken into account when conducting public meetings and outreach.
Aside from the vibration limits adopted for a specific project, general procedures—including building preconstruction condition surveys, clearly specified agreements between stakeholders, and monitoring during construction—are generally accepted procedures to follow to ensure protection of historic buildings. Stakeholders should also involve both experienced preservation professionals and engineers, especially in critical situations where the risk of damage is higher and/or the possibility of mitigating it after the fact is less acceptable.
5. TRANSPORTATION AGENCY SURVEY
The research team conducted a survey of state departments of transportation, other public agencies, and acoustical consultants to collect and synthesize successful practices currently in use. A memo summarizing the results of the survey and a copy of the survey are contained in Appendix B. A brief summary of the survey is provided below.
The following is summary of participants that were approached for the survey.
State DOTs. State DOT staff members were contacted through the membership lists of the American Association of State Highway and Transportation Officials (AASHTO) Highway Subcommittee on Construction and the Transportation Research Board (TRB) Committee on Transportation Noise and Vibration (ADC40).
Transit Agencies. The American Public Transit Association (APTA) did not respond to requests for assistance in contacting members. The research team contacted individual agencies throughout the country.
Turnpike and Toll Road Authorities. Thirty-eight members of the International Bridge, Tunnel and Turnpike Association were contacted.
Federal Highway Administration (FHWA). Stephanie Stoermer at FHWA was contacted. She did not identify any staff in FHWA Division Offices that would be appropriate to contact.
State Historic Preservation Officers (SHPOs). The research team contacted SHPO staff members through the membership list of the National Conference of State Historic Preservation Officers.
Members of TRB ADC40 Committee on Transportation-Related Noise and Vibration. Members of ACD40 were contacted through the ADC40 membership list.
Members of TRB ADC50 Committee on Historic and Archaeological Preservation in Transportation. The research team contacted members of ACD50 through the ADC50
membership list.
14
The research team used the on-line survey service Survey Monkey to implement the survey. The survey was distributed via e-mail and asked respondents to reply within 2 weeks.
5.1 Survey Response
Response Numbers
The following is a summary of the survey responses:
-
506 requests sent
-
138 total responses received (27.9%)
-
59 participants completed the entire survey (22.7%)
-
6 consultants
-
45 State DOTs
-
34 unique states (several states had multiple responses)
-
5 SHPO staff
-
2 Canadian provinces
The survey results indicate that none of the responding agencies has formal processes (i.e., adopted guidelines or official policy documents) in place for evaluating the potential effects of construction vibration on historic buildings. California appears to have done the most work in this regard with the development of a guidance manual on the effects of construction vibration, including a discussion of historic buildings.
In general, if the issue arises, the agencies’ approach varies depending on the perceived and actual sensitivity of the historic building, the nature of the construction vibration (i.e., impact pile driving vs. grading), ground conditions, and the level of concern by the public and public agencies. Although many respondents offered elements of good approaches, no single best approach was obtained from the survey results. The survey identified several case studies that are discussed later in this report. The details of the survey can be found in a summary memo in Appendix B.
5.2 Follow-Up Calls to Survey Respondents
The research team called selected survey respondents to obtain additional information. The respondents directly contacted represent both the historical preservation side of the issue as well as the engineering side. The information obtained in this follow-up effort provided more insight into the process and procedures that State DOTs follow to protect historic buildings from construction vibration damage.
In many cases individuals who were contacted had either not been in their position a long time or worked in a state where the issue does not come up frequently (e.g., less populated states and/or states without large urban areas). In spite of that, the research team was able to obtain useful information. A very informative conversation was conducted with a preservation specialist in New Mexico who has worked in this area for many years and was involved in evaluating the impacts on very old structures in historic monuments. Not only did the preservation specialist tell us of several projects, but she also provided useful contact information for engineers with
15
more specific details. In addition, the specialist provided a few more reference documents that had not been discovered in the literature search.
The research team contacted 16 survey respondents by phone, interviewed them using a focused set of questions, and then discussed specific examples in which historic buildings were considered to be potentially impacted by a transportation construction project. The research team was provided with examples of project documentation, including MOA’s, specifications, and technical studies.
In summary, the responses obtained in the follow-up calls were as follows:
-
Most states address historic buildings and protection from construction vibration damage on a case-by-case basis.
-
The issue generally arises during an environmental review process and is identified by the SHPO during the Section 106 process.
-
In some instances, the issue is raised by the public or a building owner.
-
Generally, the type of construction (e.g., blasting, pile driving, use of heavy construction equipment) and its proximity to historic buildings are what determines whether a building is considered to be potentially impacted by the project.
-
A consultation between SHPO and DOT engineers is used to determine the potential for impact and what contract requirements are necessary. Occasionally, SHPO has disagreed with DOT staff.
-
When necessary, but infrequently, stakeholders bring in an expert to assist to determine a building’s susceptibility and what protection is needed, including setting vibration limits and developing a monitoring plan.
-
Where a building or group of buildings is determined to be possibly impacted by vibration, the procedure adopted generally requires vibration monitoring at a minimum.
-
In some instances, a visual pre- and post-construction survey has been required to document the existing condition of the building (specifically existing cracks).
-
Where vibration limits are set in contract bid documents, the type of construction is typically considered in setting the limits.
-
In some instances, where vibration limits on the low side of the range were adopted, it was determined before or during construction that the existing ambient vibration was greater than the vibration limit. This resulted in an increased vibration limit. This situation could have been avoided by measuring ambient vibration prior to setting the contract limit.
-
Some contracts have included a “stop work” order when vibration limits are exceeded. However, this appears to be the exception rather than the rule.
6. CASE STUDIES
The research team selected several candidates for cases to illustrate the various aspects of the process of protecting historic buildings from construction vibration damage. The possible cases were drawn from the research team’s project experience and from project information obtained in the literature review. Unfortunately, the agency survey and follow-up calls produced no cases with sufficient information to be included. Although agency staff with whom we spoke were always willing to help, the people who were directly involved with a project had often left the
16
agency or retired. Another challenge for the research team as well as agency staff is the availability of project files, which after more than a few years are no longer easily accessible.
Once the cases to be included were selected, a major challenge faced by the research team was locating persons who were directly involved in each of the projects to provide relevant information even for those projects in which a research team member participated. In many cases, one agency staff member is involved in the planning phase, another is involved during the engineering phase, and a third may be involved during construction. Another reason for the fragmentation of information is that very few projects result in a summary report on their completion. One case (Doyle Drive) is an exception to the rule and provides a fairly complete picture of the process from beginning to end.
-
The main aspects illustrated by these cases are:
-
Identification of potentially affected historic buildings.
-
Importance of preconstruction survey of affected buildings.
-
Determination of a building’s susceptibility to vibration damage.
-
Selection of vibration limits to be imposed during construction.
-
Mitigation by monitoring to control the level of vibration at the building.
-
Supplementary vibration mitigation measures.
-
Success of the mitigation measures in preventing damage.
6.1 Case Study #1: Sacramento Railyards Central Shops (Carman, et al.)
We include this project as a case study even though the main concern was not construction vibration, but, rather, long-term vibration from freight railroad activity. We include the project because the issues were similar to those encountered when dealing with construction vibration and historic buildings and because the approach taken to determine an appropriate vibration criterion was innovative and informative.
The Railyards property, adjacent to the Amtrak station in Sacramento, contains eight massive, historic masonry buildings originally used to construct and maintain locomotive engines. The buildings are referred to as the Central Shops. The Railyards property is adjacent to the current Amtrak Station and was the western terminus of the First Transcontinental Railroad when the Central Pacific Railroad connection with the Union Pacific Railroad was completed in 1869. The Central Shops Historic District is a City of Sacramento historic and cultural resource. The city is in the process of nominating the district for listing in the NRHP.
The Intermodal Transit Facility Track Relocation Project on this site involved relocation of the existing railroad tracks that carry freight and Amtrak passenger trains. The track relocation, just recently completed, brought the tracks within 32 feet of the Central Shops. These buildings are all constructed of multi-wythe brick masonry with lime mortar joints. In general, the masonry walls are in a deteriorated condition due to weathering over many decades, and, in part, foundation settlement. Some building walls have been repointed and are in relatively good condition.
The environmental impact study performed as part of the Environmental Impact Statement (EIS) for the Sacramento Railyards Project recommended investigating vibration from freight operations and its potential effect on the Central Shops. The recommended criterion of the
17
Federal Transit Administration (FTA) to avoid damage to older buildings “extremely susceptible to vibration” is 0.12 inches/sec. Based on a detailed analysis of expected vibration levels from trains operating on the new tracks, the project’s vibration consultant recommended that a vibration mitigation measure be implemented underneath the new tracks to reduce vibration. The recommended vibration mitigation measure was an underlayment of tire-derived aggregate (shredded motor vehicle tires) below the track. However, because of engineering concerns involving flammability, this mitigation measure was not implemented.
The project’s vibration consultant predicted vibration from freight traffic to be in the range of 0.13 to 0.32 inches/sec under a worst case scenario of “severe wheel flats” on freight rail cars. Because the predicted vibration exceeded the FTA criterion of 0.12 inches/sec, there was concern. The engineers recommended further investigation with more detailed analyses and site specific measurements in the project’s design phase.
During the project’s design phase, the owner engaged a structural engineer to evaluate the condition of the buildings and the effect of future train vibration. The engineer concluded from a visual review that even though the maximum predicted vibration exceeded the FTA criterion, the vibration was not expected to cause any risk to the existing masonry walls (structural or cosmetic), with the exception of one building (Car Shop), which had a large cantilevered wall (i.e., unsupported laterally). Figure 2 shows the outside of the Car Shop’s unsupported wall.
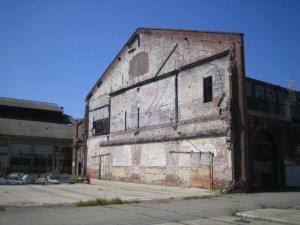
The structural engineer conducted a detailed finite element analysis to evaluate the Car Shop wall and its susceptibility to ground vibration. The vibration consultant made additional vibration measurements for freight and passenger trains and characterized the site-specific soil conditions at the Car Shop. The additional data from measurements were used to refine the predicted vibration levels expected at the base of the cantilevered wall of the Car Shop.
18
The structural engineer used the refined vibration predictions as input to the finite element model to determine maximum stresses and strains in the wall at critical points. The structural engineer was able to evaluate whether the strains from vibration were a structural risk by considering strains from wind loading. Using the finite element model, the structural engineer simulated wind loads the wall would have been subjected to over its lifetime based on historical data of nearby recorded maximum wind loads. Comparing strain levels in the wall due to freight train vibration with strain levels due to recorded maximum wind loading, the structural engineer concluded the wall was stable for the anticipated train-generated vibrations predicted for the site once remedial structural work was done to meet the local building code.
This case demonstrates that it is possible through site-specific and detailed engineering analysis to determine with more certainty the effects of vibration on an historic building and to develop a building-specific criterion to protect the building. Although this case is unique, it demonstrates the steps and procedures that can be taken (possibly on a lesser scale) to arrive at a more definitive assessment for a specific building, rather than taking a more generic approach.
6.2 Case Study #2: Cypress Lawn Cemetery – Entrance Archway and de la Montaña Mausoleum
This case involved the construction of a new subway line to the San Francisco International Airport for the Bay Area Rapid Transit (BART) system. The right-of-way used for the construction was the original railroad alignment for Southern Pacific Railroad circa 1870, which provided rail access to San Francisco during the late 19 th Century. The old railroad alignment, which fell out of use during the 1940’s, passes through several cemeteries developed over the last century on both sides of the right-of-way. Although the cemeteries had encroached on the alignment over time, California law gave them certain easement rights requiring BART to reach an agreement with the cemeteries on several issues.
Most of the cemeteries, including Cypress Lawn Cemetery, are a NRHP historic district. Cypress Lawn has 21 historic resources, including a chapel, columbarium, and numerous tombs and mausoleums. The portion of Cypress Lawn Cemetery through which the subway was built is considered to be one of the last grand rural garden cemeteries built in the West and is the final resting place for such luminaries as Charles De Young (newspaper), Charles Crocker (railroad), George Hearst (newspaper), and Wyatt Earp (lawman).
Two historic buildings, an historic archway, and an historic family mausoleum were relatively close to the subway tunnel construction, causing concern they might be damaged by construction vibration. As a result of the Memorandum of Understanding (MOU) between BART and the various affected cemeteries, including Cypress Lawn, vibration monitoring during construction was conducted at several buildings and structures. The Cypress Lawn entrance archway (Grand Gateway) and the de la Montaña Family Mausoleum were among those monitored.
Subway construction involved a cut-and-cover excavation technique called soil mix wall. The soil mix wall (one on each side of the subway box) provides support for the surrounding soil during excavation of the trench within which the concrete box for the subway structure is built. A soil mix wall is constructed by drilling several rows of holes into the ground and inserting soldier piles (H-shaped beams) in each of the holes. The holes are then filled with a soil mix (cement-based binder slurry). Once the soil mix has hardened, forming a wall underground, the soil in between the two walls is excavated and the concrete structure is constructed between the two walls. All of these activities generate vibration.
19
The most significant vibration generated during the subway box construction occurred during what is called “bond break” between the soldier piles and the soil mix, 24 hours after pouring of the soil mix and prior to its reaching full hardened strength. The bond break is achieved by vibrating the piles with the same vibratory hammer that is used to drive them a short distance into the ground below the drilled hole. The purpose of the bond break is to minimize the vibration generated when the piles are later extracted. Extraction of the soldier piles allowed the contractor to reuse the piles in subsequent sections of the construction. Excavation also generated vibration levels that were substantial, as did the movement of equipment used for excavation when in close proximity to the monitoring locations adjacent to the two historic structures.
The contract construction specifications developed by BART specified that cemetery structures and buildings in weakened condition (as determined by a preconstruction visual survey) would have a vibration limit of 0.08 inches/sec. For structurally sound buildings, the vibration limit was specified as 0.2 inches/sec. The archway is a granite masonry structure constructed in 1892 and one of the earliest examples of Mission Revival-style architecture. The vibration limit adopted for the archway was 0.08 inches/sec based on its age, even though it was a well maintained structure. As agreed to in the MOU and as a protective measure, BART had the archway repointed at its expense. BART documented the condition of all potentially affected structures with still and video photographs prior to start of construction.
Figure 3 shows the archway and a seismograph used for monitoring vibration. In addition to the seismograph at the base of the archway, there was an alarm system with warning lights (yellow for caution and red for exceedance) and an audible alarm. The warning system provided a direct feedback to the equipment operators when vibration limits were being approached or exceeded and allowed equipment operators to adjust their activity. After an initial learning stage of trial and error, the system became a reasonably successful tool to control vibration.
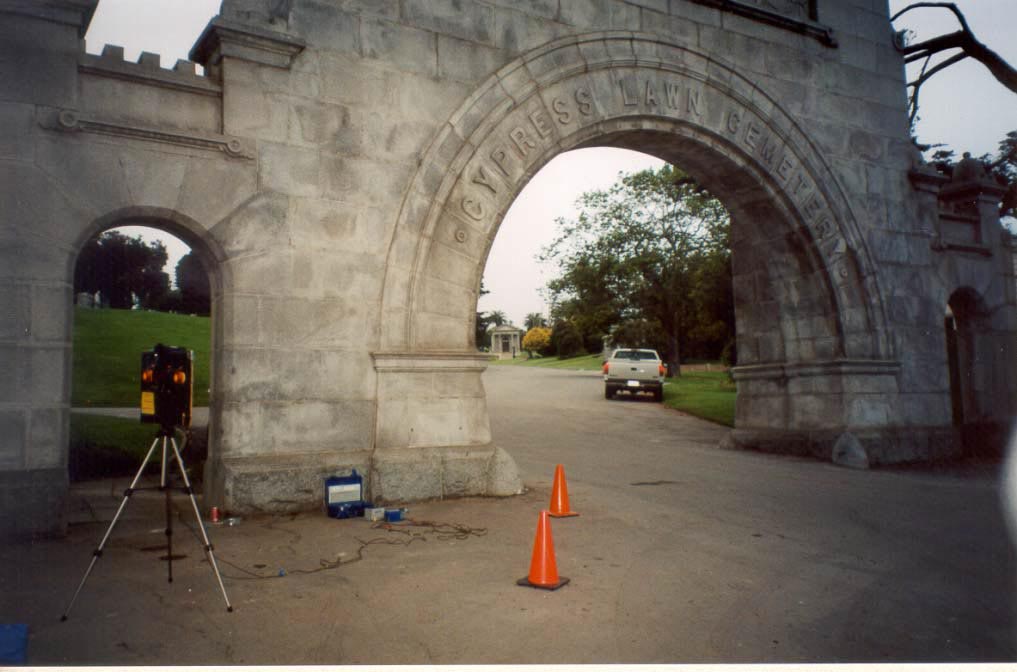
20
The implementation of the alarm system was somewhat unusual, but the cost was inconsequential because the seismograph was configured to allow for an alarm. The alarm’s main advantage was that it allowed for real-time feedback to the equipment operator, thus increasing the chances of successful compliance with the vibration limit. In general, the response of the equipment operator to having an alarm was positive, and he was able to modify the activity of his equipment to stay within the vibration limits.
Although not implemented on this project, current seismograph technology allows phone numbers to be dialed every time a threshold is exceeded (i.e., either warning or limit). Generally, such a call would be made to at least the Resident Engineer or a representative who would then assess the situation and decide what changes in activity or equipment were necessary.
Vibration levels measured at the archway during construction of the BART project ranged from 0.09 to 0.12 inches/sec. The higher vibrations occurred during excavation and by movement of a small Caterpillar approximately 15 feet away from the archway. Although these levels were above the limit of 0.08 inches/sec, engineers found no damage to the archway during inspections during and after construction.
Figure 4 shows the de la Montaña Mausoleum. The mausoleum was constructed in 1907. The lower part of the structure is steel framed with sandstone cladding, while the upper part is a stone finial that sits on four stone arches atop four large granite blocks. At the time of BART subway construction, the de la Montaña Mausoleum was in a deteriorated condition due to lack of maintenance.
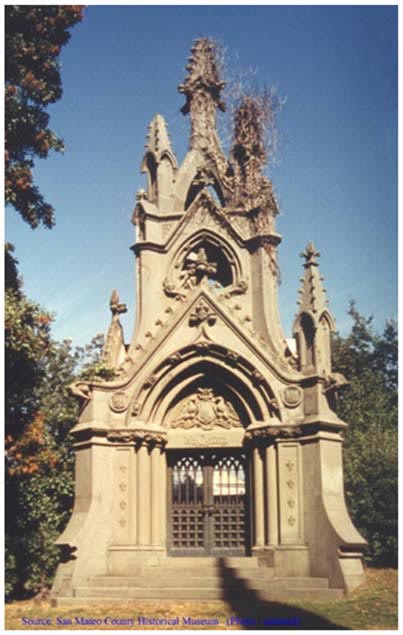
Based on the structure’s age and condition, the vibration limit for the mausoleum was set at 0.08 inches/sec. To avoid any serious damage to the mausoleum and to protect its structural integrity, BART had the contractor install temporary interior shoring using scaffolding to support the structure at critical points. A structural engineer re-evaluated the vibration limit of 0.08 inches/sec and determined the limit could be raised to 0.138 inches/sec with the interior supports.
21
In addition, the contractor was not allowed to break bond between the slurry wall and soldier piles in a “no pull zone” closest to the historic structures. Construction vibration levels measured at the mausoleum ranged from 0.09 to 0.11 inches/sec (less than the vibration limit of 0.138 inches/sec) during the bond break of piles in the soil mix wall at the closest point to the mausoleum (approximately 135 feet away).
In spite of these precautions, Cypress Lawn filed a lawsuit after construction was complete claiming construction vibration damaged the de la Montaña Mausoleum. BART retained an engineering team, which analyzed the damage in two ways:
1) The team reviewed the preconstruction videos and photographs, including historic photographs in local archives, and found that all the claimed damage existed before construction.
2) The engineers performed calculations comparing construction vibration energy and frequency content with that from earthquake ground shaking. They found that earthquakes in 1957 and 1989 had peak velocities 20 and 120 times greater, respectively, than the vibrations caused by the construction. They found that the energy (measured as a function of ground velocity squared) of the 1957 and 1989 earthquake ground shaking was 85 and 10,000 times greater, respectively, than the energy generated during the soldier pile extraction and bond breaking. The earthquakes were sufficient to have caused much of the observed stone displacement.
The lawsuit was settled during arbitration in accordance with the findings that the BART construction did not cause damage to the mausoleum.
This case demonstrates several aspects of the process of evaluation, agreement, monitoring and settlement of issues with construction vibration and historic buildings and structures. Although BART had to defend its actions and the activities of its construction contractor, it was able to show that it had taken reasonable and appropriate efforts to avoid damage to the historic cemetery buildings. The preconstruction photos and videos taken by BART were a critical component in proving that the BART construction had not damaged the mausoleum.
This case illustrates how a warning system could be provided as a real-time interface between the vibration monitoring and the contractor, thus facilitating better control over vibration and better adherence to vibration limits. A warning system is not a commonly used measure and would usually be implemented only when high levels of vibration were expected to occur during construction in close proximity to the historic building. However, the expense of an alarm system is minimal, and a system can easily be constructed from commonly available material. The other unique feature on this project was scaffolding installed inside the mausoleum structure. This approach is uncommon, but could be used where deemed necessary for protection and minimizing the risk justifies the cost. This case also emphasizes the importance of a preconstruction survey.
6.3 Case Study #3: Gipfel Brewery Building, Milwaukee (HNTB Corporation and Wilson, Ihrig and Associates)
An evaluation was prepared by a vibration consultant for the Wisconsin DOT and the City of Milwaukee for the effects of freeway demolition (Park East) on three historic buildings in
22
downtown Milwaukee. One of the buildings (Gipfel Union Brewery) was built in 1853 in the Federal style and was the oldest brewery building still standing in the city, although Gipfel ceased operation in the 1890s. The Gipfel building was designated a city landmark in 1985 and determined by the Wisconsin Historical Society to be eligible for the NRHP in 2000. The Gipfel building was the only remaining resource from the historic Kilbourntown, one of the original communities that became Milwaukee.
The Gipfel Union Brewery (a three story brick building) was 100 feet from the nearest freeway structure to be demolished. A second building (Gugler Building) in the same area was built in 1896 in the German Renaissance Revival style. The Gugler Building (composed of a one story and a two story brick building) was 95 feet from the freeway structure. The third building (WEPCO Switch House) was built between 1903 and 1912. The Switch House (a two story brick building with a reinforced concrete foundation, ca. 1942) was the city’s primary source of electricity until the middle of the 20 th century. The Switch House was located 20 feet from the freeway structure.
The Park East Study (HNTB-) contains an extensive review of vibration criteria. In the evaluation of the buildings and derivation of recommended criteria, the vibration consultant used the classification system for buildings contained in the British standard BS 7385, Part 1. Under this standard, each structure is rated according to four categories: the type of construction, the foundation, the soil, and political importance. As a result of the analysis, the consultant recommended a limit of 0.15 inches/sec for the Gipfel Union Brewery, 0.40 inches/sec for the Gugler Building, and 1.2 inches/sec for the WEPCO Switch House.
The activity expected to generate the greatest vibration was the dropping of large portions of freeway structure during demolition. According to the project manager, vibration monitoring was conducted during demolition of the freeway structure with no apparent damage to the buildings. Unfortunately specific vibration monitoring data were not available.
An interesting if sad footnote to this story, according to a 2009 newspaper article, was that, unrelated to the Park East project, the City of Milwaukee deemed the Gipfel building unstable and ordered the building owners to raze the building because it posed a threat to the safety and welfare of the public. The owners previously had moved it from its original location one block away with the intent of incorporating the brewery building into another development. However, the lead developer of the new project rejected this plan. The building owners still had hopes of saving the building, but, unfortunately, time ran out and the building was demolished in 2009. Figure 5 shows the building in the process of being moved.
This case demonstrates that by using an established classification system, it is possible to tailor the specified vibration limits to individual buildings. It also provides an example of how a smaller building could be moved to save the building if no other means were available to avoid damage, even though removing the building from its original surroundings and historical context might nullify the building’s historical status.
23
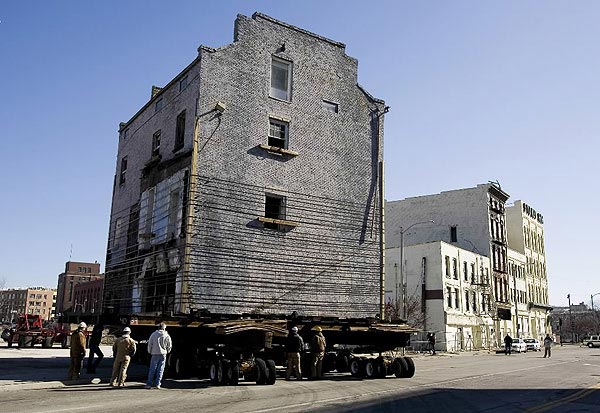
6.4 Case Study #4: Fraunces Historic District, New York City (Esrig and Ciancia)
Although this was not a transportation project, it involved construction adjacent to historic buildings and provides a good example of steps used in evaluation and determination of vibration limits. The structural engineers (Esrig and Ciancia) were retained to address the effects of vibration from construction of a 29 story office tower adjacent on buildings in an historic district in lower Manhattan. The construction of the office tower involved blasting of the underlying granite and pile driving.
The potentially affected buildings in the historic district were constructed between 1820 and 1970 on fill placed in the area during the 17 th Century. The historic buildings included 16 three and six story buildings located on one city block. The engineers indicated in their investigation that some of the buildings had undergone extensive renovations. Figure 6 shows Fraunces Tavern, one of several historic buildings within the Fraunces Historic District.
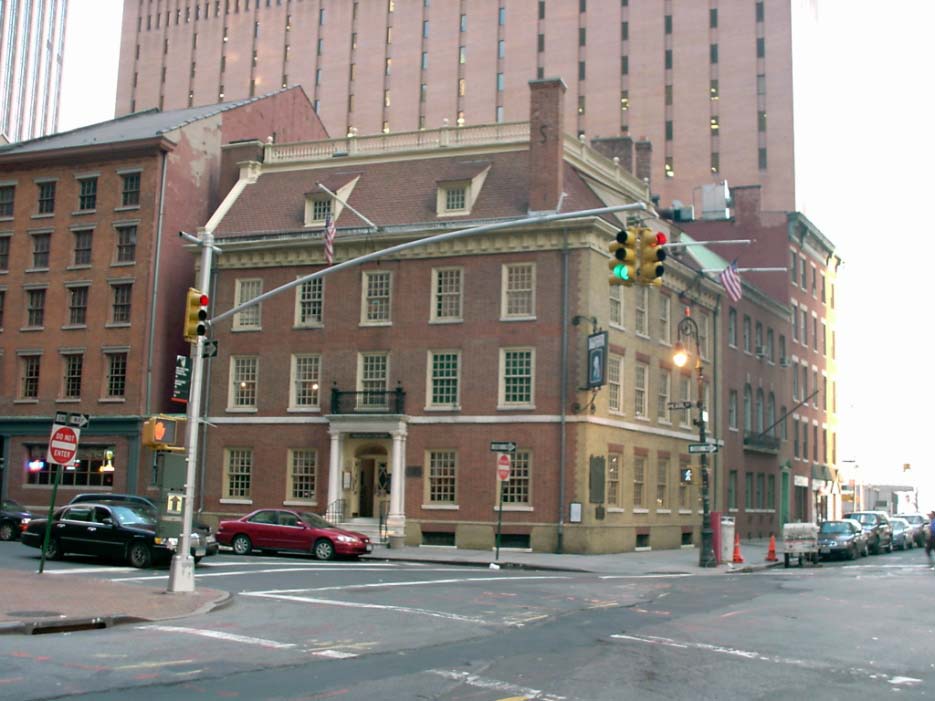
As part of an earlier renovation of one of the buildings, test pits were dug in some basements to determine the foundation type and condition. The engineers recommended grouting the soil to
24
provide additional support for the foundation. For the office tower project, characterization of the soil was determined by digging test pits within some of the historic building’s basements. The soil type was used to help determine appropriate construction vibration limits.
The engineers were tasked with arriving at permissible vibration levels and with other issues concerning movement of temporary restraining systems and movement of the buildings. The engineers list various limits for peak particle velocities ranging from 0.2 to 1.0 inches/sec. The New York City Department of Environmental Protection limit was 0.2 inches/sec for old brownstone buildings, according to the journal article. The engineers decided to recommend a limit of 0.5 inches/sec with the requirement that if either building movement or additional cracking during blasting and/or pile driving occurred, the limit would be reduced.
Monitoring of ambient conditions before construction indicated vibration that was generally less than 0.1 inches/sec, with only one level that reached 0.2 inches/sec. Monitoring during pile driving detected a maximum vibration level of 0.18 inches/sec. Based on visual inspection, it was determined that the historic buildings showed no measureable signs of distress or movement during the vibratory driving of the sheeting (sheet piles). Monitoring performed during blasting indicted vibration of less than the limit of 0.5 inches/sec, and no measureable distress was evident.
6.5 Case Study #5: Fort Point – San Francisco
After the Loma Prieta earthquake in 1989, the Golden Gate Bridge District evaluated the vulnerability of the Golden Gate Bridge in the event of an earthquake of even greater magnitude occurring in the vicinity of the bridge. The Bridge District’s consultants determined that there was a risk of major damage if an earthquake with an epicenter near the bridge and a magnitude of 7.0 occurred, and that the bridge could collapse if the magnitude were 8.0. The consultants also determined that retrofitting the bridge to improve its resistance to an earthquake would be less expensive than replacing the bridge after a major earthquake.
Directly underneath the south end of the bridge is the historic Fort Point a masonry structure built in 1854. Figure 7 shows the inside of Fort Point with the bridge above. The contract specification for the bridge retrofit required vibration to be limited to less than 0.1 inches/sec for continuous activities and 1.0 inches/sec for single-event activities occurring near Fort Point.
25
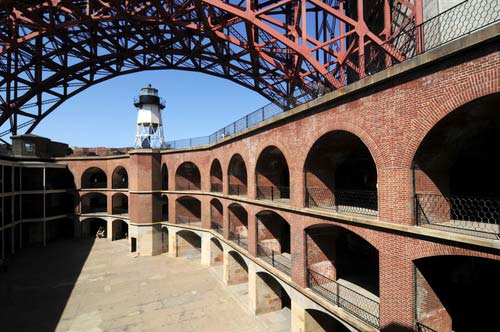
During Phase 1 remediation, cast-in-drilled-hole (CIDH) piling and pile caps were added around the perimeter of the original bridge foundation pedestals. The interface of new concrete to existing concrete was strengthened with post-tensioning of monostrands, clamping the new footings to the pedestals of the existing foundations. The existing grade beams between the foundation pedestals were also substantially strengthened, and additional grade beams were constructed. After the existing towers supporting the bridge were removed, the second stage of the foundation retrofit proceeded. First, the remaining upper portions of the existing pedestals were demolished. Then, new upper pedestals were constructed and closure pours placed to incorporate these elements into the entire foundation system. The erection of a new tower followed.
The most visually dramatic Phase 1 work was the complete removal and replacement of the four steel support towers with footprints of 50 feet by 75 feet and heights of up to 150 feet. The contractor sequentially replaced the existing towers with new ones that very closely imitate the appearance of the original towers. Each of these construction activities involved the generation of groundborne vibration.
Vibration monitoring was performed inside and outside the fort at points closest to the construction activity. Ambient monitoring indicated vibration levels that were quite low (i.e., less than 0.01 inches/sec) except during isolated events that could be attributed to people walking in the vicinity of the seismograph. Ensuring that non-construction vibration from activity of a local nature, which occurs next to the vibration sensor, is properly identified and not erroneously attributed to construction activity is always an issue for vibration monitoring. The person performing the monitoring must often query building occupants, review construction activity records, or undertake other analysis to determine the cause of the vibration.
The most significant activity for vibration was the driving and removing of sheet piles. The maximum recorded vibration for sheet pile activity was 0.17 inches/sec. Other vibration generating activities involved drilling for CIDH piles and excavation for new foundations at the towers. For the drilling, the maximum vibration recorded was less than 0.09 inches/sec.
26
6.6 Case Study #6: Historic District, New York City (Hammarberg, et al.)
Hammarberg and others involved in this project are structural engineers engaged to assess two construction projects in New York City that were in close proximity to historic buildings. The engineers recommended vibration limits and developed detailed plans for monitoring prior to and during construction.
In a paper published after the projects were complete, the engineers explain in clear terms the fragile nature of historic structures, which was typically due to the brittle materials (brick masonry, terra cotta, and plaster) and construction methods. The difficulty in minimizing construction impacts on historic structures, especially in urban areas, is explained. In addition to the effects of vibration on buildings, this paper also discusses the effects due to excavation adjacent to older buildings and the need to underpin to prevent soil settlement. Soil settlement caused by nearby excavation is a phenomenon similar to vibration caused settlement and equally as important.
Hammarberg distinguishes, as do most others, the difference between structural damage (that which affects the capacity of the primary or secondary load bearing members in the building) and cosmetic damage (that which affects the appearance of the building, but not the structural capacity). As the paper notes, the envelope of the building is the most likely to suffer cosmetic damage due to cracking of exterior finishes and growth of existing cracks, especially in older buildings that are often heavily ornamented. The weakening of building elements as a result of the aging process caused by weathering, temperature variation, freeze-thaw and long-term settlement of the structure is also a concern. A building that has suffered from what might be called the ravages of time is more likely susceptible to additional damage.
The paper provides a good description of the nature of construction vibration and how it interacts with structures, and the paper lists the most common types of vibration producing construction activity. Vibratory installation of sheet piles, pile driving, and vibratory compaction of soil are examples of long-term activity. Of a more short-term nature is the vibration associated with blasting and dynamic soil compaction.
In a discussion on setting vibration limits, the authors indicate that the generally adopted values come from empirical data that address vibration source, soil type(s), and condition of the structure and that there is no single, widely recognized standard for allowable vibration limits for historic structures. In spite of this lack of standards, the authors say it may be possible to predict a particular project’s “probable damage” to an historic structure adjacent to construction when determining a “rational” vibration limit. Hammarberg indicates that the most appropriate standards for protection of historic structures are the standards that were developed for the protection of older, damaged or, in other words, more vulnerable structures.
The authors indicate that the Swiss, German and Central/Artery Tunnel (Boston) vibration standards were found to have prevented damage during construction in urban areas. In New York City the limit for historic buildings is 0.5 inches/sec, which Hammarberg et al. point out may not be sufficient to avoid damage to fragile historic buildings. Hammarberg discusses the need to determine a balance between providing safe vibration limits without precluding cost effective construction. Of importance, the authors note the vibration limits derived from research
27
conducted by the U.S. Bureau of Mines on the effects of blasting on residential structures are the least appropriate standards for protection of historic structures.
Hammarberg emphasizes the importance of requiring a preconstruction survey of the historic building that includes:
-
Description of the building
-
Type of building construction
-
Age of construction
-
Foundation type
-
Condition of the building
-
Location, width and orientation of visible defects and/or cracks
-
Location of loose material
-
Location of previous repairs
-
Distance to construction operations.
The mitigation measures applied to the building could include securing loose materials prior to construction to keep them from falling and supplemental support for equipment that might sway. Routine visual surveys during construction are recommended to observe if changes are occurring and to evaluate if new damage has occurred. The most important mitigation measure is to monitor vibration using proper instrumentation, according to Hammarberg. Existing cracks should be monitored to observe whether changes occurred during adjacent construction activity. A simple visual or electronic gage can serve as a monitor.
Hammarberg present two case studies involving historic buildings in New York City dating from the late 19 th century. The unique features of two buildings (early sky-scrapers of 15 and 21 stories) in the first case that make them susceptible to vibration are explained in detail. An interesting recommendation was to include a gas leakage monitoring system.
The second case study involved an historic district of buildings dating from the early 19 th Century. In this study, the foundations of buildings in this district were determined to be particularly susceptible to vibration damage due to their construction with loose material (stacked stone and rubble). Four vibration threshold levels were recommended by the engineering team. With each level, there were recommended reporting, engineer action and contractor action requirements, with the latter ranging from no action to cessation of all work.
6.7 Case Study #7: Presidio Buildings – Doyle Drive, San Francisco (ICF International)
Doyle Drive is located in the Presidio, in the northern part of the City of San Francisco. Doyle Drive, the southern approach of State Route 101 to the Golden Gate Bridge, is l.5 miles long with six traffic lanes. Doyle Drive passes through the Presidio on an elevated concrete viaduct (low viaduct) and transitions to a high steel-truss viaduct (high viaduct) as it approaches the Golden Gate Bridge toll plaza. The Presidio was designated a National Historic Landmark (NHL) in 1962. In the 1993 NHL update, Doyle Drive was determined to be a contributing element to the Presidio National Historic Landmark District.
28
A vibration study was conducted to evaluate the potential for damage to historic buildings at the Presidio that could result from construction activity (ESA 2004). With regard to potential damage criteria, the report states:
“[A] ground vibration PPV of 2 mm/sec (0.08 inches/sec) would be a conservative, but appropriate limit for the historical buildings that are more susceptible to damage, including those in a poor structural condition and those of masonry construction. The buildings in the Main Post area of the Presidio are mostly of masonry construction and would therefore fall into this category. In addition, visual observation of the buildings under consideration in this study indicates some existing differential settlement and cracks in the facades of the brick buildings. However, most of the other historical buildings in the Presidio are wood-framed structures which are substantially less susceptible to damage from vibration. For most of these buildings, a higher PPV of 5 mm/sec (0.2 inches/sec) would be an appropriate, conservative limit for construction vibration. The exterior facades of some of these wood-framed buildings (such as the Mason Street warehouses) are in a poor condition, although it is understood that these buildings are structurally sound.”
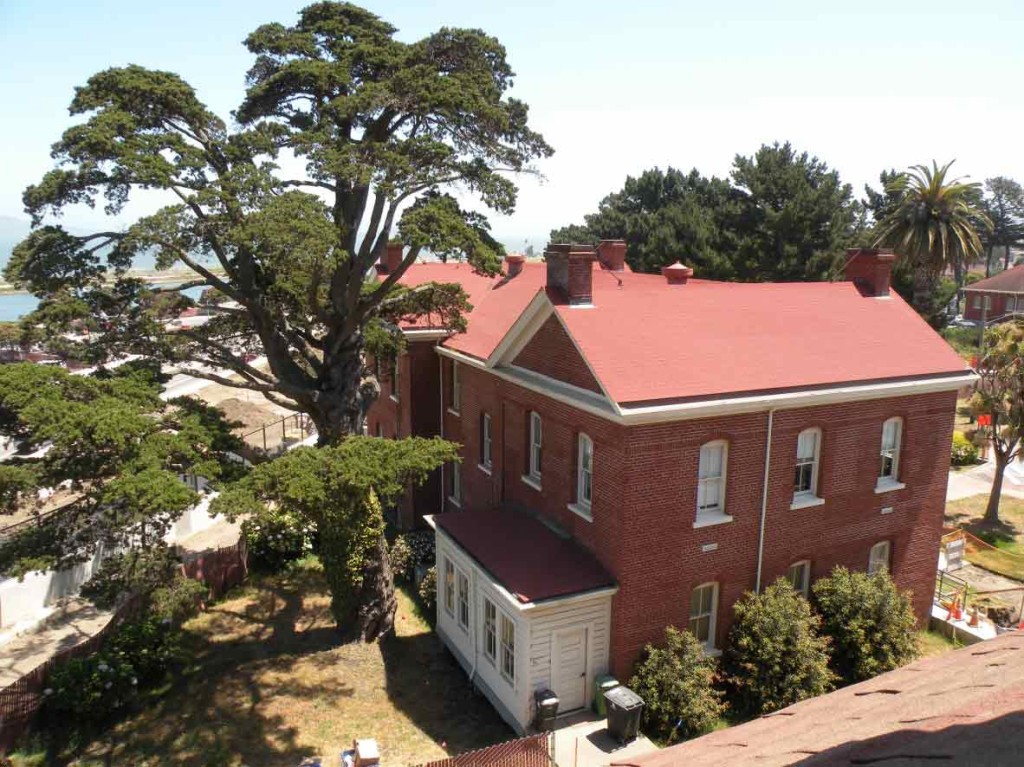
Impact pile driving and dynamic compaction were identified as the activities that would typically generate the highest vibration level. Pile driving was required along much of the route. The tunnel road structure needed to be supported by piles, and sheet piling was installed during the excavation of one the tunnels. Dynamic compaction of the road surfaces using vibratory rollers was also required along much of the route.
29
The report indicates that the expected worst-case ground vibration velocity from impact pile driving would be less than 0.08 inches/sec PPV at a distance of 200 feet and less than 0.2 inches/sec PPV at 100 feet, even allowing for soil conditions that tend to assist the vibration propagation.
The report states that alternate means of pile driving would be used within a 200-foot buffer zone around the Presidio’s historic buildings. These alternate measures would include vibratory piling, use of drilled piles, and pile jacking (where the piles are pressed into the ground by means of a hydraulic system, resulting in far less vibration). The report further states that although vibratory pile driving generally produces less vibration than conventional impact pile driving, vibratory pile driving could still generate ground vibration levels exceeding 0.08 inches/sec within about 100 feet and could exceed 0.2 inches/sec within about 50 feet. Where drilled piles are utilized because of vibration considerations, the report recommends that the steel casings be put in place by jacking rather than a standard vibratory process.
The report states that vibratory rollers can generate relatively high levels of ground vibration and that the maximum expected levels range up to about 0.08 inches/sec at a distance of 70 feet. The report further states that no damage would be expected due to a medium-to-heavy roller at distances greater than approximately 70 feet.
The report states that there would be minimal risk of damage to the historic buildings within the Presidio due to construction-induced building vibration provided that appropriate vibration limits are incorporated in the construction contracts and the vibration levels are controlled to within those limits by utilizing alternate demolition and construction procedures where necessary.
The viaducts are in close proximity to historic buildings; therefore, the method of demolition will not involve simply dropping the structures to the ground. Alternative approaches include lowering demolished viaduct structures by crane or the use of earthen cushions. If earthen cushions are used, their effect in reducing vibration will first be evaluated in less sensitive areas of the project site but within the project’s archaeological APE (the area in which there will be ground-disturbing activity). To reduce potential vibration impacts on historic buildings from breaking up reinforced-concrete structures on the ground, the components will be placed as far as is feasible from the buildings, but still within the archaeological APE, before they are broken up.
The vibration levels will be monitored. If blasting for either demolition or construction is permitted, the blast weights and blast design will be based on achieving compliance with conservative ground-vibration limits at the closest buildings. The Built Environment Treatment Plan, an addendum to the multi-agency MOA, adopted the conservative vibration limits specified in the vibration study (ICF International 2009).
The first phase of project construction is close to completion. Vibration monitoring was conducted by a vibration measurement firm retained by the construction contractor. Monitoring reports are now available. Monitoring alarms were triggered at three neighboring World War I era warehouses. However, it is understood that there were issues with ambient vibration triggering exceedance warnings, which made monitoring of construction-induced vibration difficult. There were some instances where a building’s changed condition may or may not have occurred as a result of vibration. The California Department of Transportation (Caltrans) made the decision to make repairs to the buildings rather than contest causality.
30
7. RECOMMENDATIONS
7.1 Assessment Approach
The flow chart provided in Appendix C provides a suggested guideline approach for addressing construction vibration effects on historic buildings and measures to avoid damage. This approach is a distillation of approaches based on information gathered from the literature review, the results of the agency survey, follow-up calls, and the experience of the research team.
The approach entails a screening procedure assuming a conservative APE due to vibration, use of conservative thresholds for potential damage, and simple vibration prediction methods indicated in FTA’s Transit Noise and Vibration Impact Assessment (Federal Transit Administration 2006) and Caltrans’ Transportation- and Construction-Induced Vibration Guidance Manual (California Department of Transportation 2004). The FTA’s simple prediction method is based on the following equation (check the original document for accuracy of the following):
PPVequip = PPVref x (25/D)^n
where:
PPV (equip) is the peak particle velocity in in/sec of the equipment adjusted for distance
PPV (ref) is the reference vibration level in in/sec at 25 feet
D is the distance in feet from the equipment to the receiver
n is the attenuation exponent
n = 1.5 for competent soils: most sands, sandy clays, silty clays, gravel, silts, weathered rock (can dig with a shovel)
n = 1.1 for hard soils: dense compacted sand, dry consolidated clay, consolidated glacial till, some exposed rock (cannot dig with a shovel, need a pick to break up)
Table 2 shows typical source levels reported in FTA guidance document.
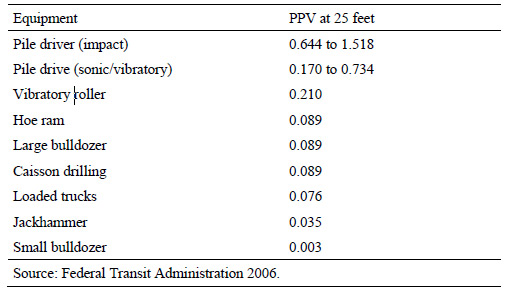
31
As an example the predicted vibration level at 500 feet from pile driving in hard soils would be
calculated as follows:
PPVequip = 1.518 x (25/500)^1.1= 0.056 in/sec
The recommended screening distance for potential vibration effects is 500 feet for all but blasting activity. Where adopted, state agencies commonly use a distance of 200 feet within which potential vibration effects from construction (except for blasting) are evaluated, although one state indicated it will consider distances of 500 to 1,000 feet in some cases. The example calculation above also shows that vibration from pile driving would typically be less than 0.1 in/sec, which is a conservative threshold for potential damage. Accordingly, 500 feet is considered to be a reasonable and conservative threshold for the screening for non-blasting sources. If blasting is involved, no maximum distance is recommended. It would be advisable to conduct the screening calculation where historic buildings will be located within a few thousand feet of the blasting.
The screening process uses thresholds of 0.2 in/sec for blasting and impact pile driving, and 0.1 in/sec for continuous vibration. These values are at the lower end of thresholds commonly used for fragile buildings. If vibration is anticipated to exceed these thresholds, then feasible measures for reducing vibration should be evaluated. These measures may include alternate construction or demolition methods, such as those described in the FTA and Caltrans guidance manuals. If exceedance of these thresholds is still anticipated, the process moves into a higher level of analysis involving a detailed evaluation of the buildings for fragility, development of more refined project-specific damage thresholds, and a more detailed vibration prediction analysis.
7.2 Research and Approach to Disseminate Research Findings
To disseminate the findings of the study, the research team recommends that notifications concerning publishing the final report on the TRB website site be sent to all of the survey respondents and all individuals who were contacted to participate in the survey (see the survey summary above). In addition, where feasible, the principal authors will give presentations on the report findings at TRB ADC40 and ACD50 winter or summer meetings.
8. CONCLUSION
This report summarizes the results of a literature search, a survey of transportation agencies, and follow-up calls with selected state DOT staff on the topic of construction vibration effects from transportation projects on historic buildings. A detailed discussion of seven informative case studies has also been provided.
There is no standard or commonly accepted approach for assessing the effects of construction vibration from transportation projects on historic buildings. Recommended vibration limits tend to vary considerably within the published literature, in national standards, and in practice. However, general procedures, such as building preconstruction condition surveys, clearly specified agreements between stakeholders, and monitoring during construction, are widely followed to ensure protection of historic buildings.
This report also provides a suggested guideline approach for addressing construction vibration effects on historic buildings and measures to follow to avoid damage. This approach is a 32 distillation of approaches based on the literature review, the results of the agency survey, follow up calls, and the experience of the research team.
Click below to see the original document in its entirety.